Murine respirovirus
Murine respirovirus, formerly Sendai virus (SeV) and previously also known as murine parainfluenza virus type 1 or hemagglutinating virus of Japan (HVJ), is a negative sense, single-stranded RNA virus of the family Paramyxoviridae,[1] a group of viruses featuring, notably, the genera Morbillivirus and Rubulavirus. SeV is a member of genus Respirovirus. The virus was isolated in Sendai (Japan) in the early 1950s. Since then, it has been actively used in research as a model pathogen. The virus is infectious for many cancer cell lines (see below) and has oncolytic properties demonstrated in animals model[2][3] and natural form of cancers.[4] SeV ability to fuse eukaryotic cells, was used to produce hybridoma cells capable of manufacturing monoclonal antibodies in large quantities.[5]
As an infection agent
SeV replication occurs exclusively in the cytoplasm of the host cell. One replication cycle takes approximately 12–15 hours with one cell yielding thousands of virions.[6]
Susceptible animals
The virus is responsible for a highly transmissible respiratory tract infection in mice, hamsters, guinea pigs, rats,[7] and occasionally marmosets[8] with infection passing through both air and direct contact routes. The virus can be detected in mouse colonies worldwide,[9] generally in suckling to young adult mice. A study in France reported antibodies to SeV in 17% of mouse colonies examined.[10] Epizootic infections of mice are usually associated with a high mortality rate, while enzootic disease patterns suggest that the virus is latent and can be cleared over the course of a year.[1] Sublethal exposure to SeV can promote long-lasting immunity to further lethal doses of SeV.[11] There are no scientific studies using modern detection methods, which would identify SeV as an infectious agent that can cause disease in humans or domestic animals.

Variable susceptibility to infection in mouse and rat strains
Inbred and outbred mouse and rat strains have very different susceptibility to Sendai virus infection: The 129/J mice tested were approximately 25,000-fold more sensitive than SJL/J mice.[12] C57BL/6 mice are highly resistant to the virus, while DBA/2J mice are sensitive.[13] C57BL/6 mice showed slight loss of body weight after SeV administration, which returned to normal later. Only 10% mortality rate was observed in C57BL/6 mice after the administration of very high virulent dose of 1*105 TCID50.[14] It was shown that resistance to the lethal effects of Sendai virus in mice is genetically controlled and expressed through control of viral replication within the first 72 hours of infection.[13] Treatment of both strains with exogenous IFN before and during viral infection led to an increase in survival time in C57BL/6 mice, but all animals of both strains ultimately succumb to SeV caused disease.[15] If a mouse survives a SeV infection, it develops a lifelong immunity to subsequent viral infections.[16]
There are SeV-resistant F344 rats and susceptible BN rats.[17]
SeV induced immunomodulation
The virus is a powerful immunomodulator; it can cause a strong immune response. However, SeV can act in both directions: it can activate or suppress the immune response depending on the type of cell and host.
The mechanism of virus induced immunosuppression
SeV is able to suppress the IFN production and the IFN response pathways as well as inflammation pathway.
Apoptosis inhibition
Sendai virus P gene encodes a nested set of proteins (C', C, Y1 and Y2), which are named to collectively as the C proteins (see the section "genome structure" below). C proteins of SeV are able to suppress apoptosis.[18] The antiapoptotic activity of the C proteins supports SeV infection in the host cells.
Interferon production inhibition
SeV can suppress cell defense mechanisms by inhibiting the interferon production. The virus prevents the stimulation of type 1 IFN production and subsequent cell apoptosis in response to virus infection by inhibiting the activation of IRF-3.[19][20][21] Two virus proteins: C and V are mainly involved in this process.
C protein contribution
Inhibition of stimulation of type 1 IFN production also occurs by suppressing the phosphorylation of IRF7 by viral C protein. The C protein interacts with IKKα serine / threonine kinase and prevents the phosphorylation of IRF7. Thus, C-protein inhibits a pathway that includes a Toll-like receptor (TLR7) and TLR9-induction of IFN-alpha, which is specific for plasmacytoid dendritic cells.[22]
V protein contribution
V protein from SeV and many other paramyxoviruses has been shown to directly bind MDA5 and inhibit its activation of the IFN promoter.[23][24] V protein can also bind RIG-I and TRIM25. This binding prevents downstream RIG-I signaling to the mitochondrial antiviral signaling protein (MAVS) by disrupting TRIM25 -mediated ubiquitination of RIG-I.[25]
Human Parainfluenza Virus type 1 (HPIV1), which is a close relative of SeV and (in contrast to SeV) a successful human pathogen, does not express V proteins, only C proteins. So, all needed functions provided by V in SeV can be provided by C in HPIV1. Thus, C and V have these "overlapping functions" because of the multi-faceted nature of host defense that can be countered at so many places, and exactly how well and where will in part explain host restriction.[26]
Inhibition of the response to interferon
SeV can attenuate cell defense mechanisms and allow itself to escape from host innate immunity by inhibiting the interferon response pathway in addition to inhibiting the interferon production. C protein of the virus suppresses the signal transduction pathways of interferon alpha/beta (IFN-α/β) and IFN-γ by binding to the N-terminal domain of STAT1.[27][28]
Inhibition of nitric oxide production due to C protein
C protein of the virus inhibits the production of nitric oxide (NO) by murine macrophages[29][30] that has cytotoxic activity against viruses.[31]
Inhibiting the inflammasome NLRP3 assembly due to V protein
V protein of SeV also suppresses the production of interleukin-1β, by inhibiting the assembly of the inflammasome NLRP3.[32]
Symptoms in animals
- Sneezing
- Hunched posture
- Respiratory distress
- Porphyrin discharge from eyes and/or nose
- Lethargy
- Failure to thrive in surviving babies and young rats
- Anorexia
Diagnosis and prophylaxis
SeV induces lesions within the respiratory tract, usually associated with bacterial inflammation of the trachea and lung (tracheitis and bronchopneumonia, respectively). However, the lesions are limited, and aren't indicative solely of SeV infection. Detection, therefore, makes use of SeV-specific antigens in several serological methods, including ELISA, immunofluorescence, and hemagglutination assays, with particular emphasis on use of the ELISA for its high sensitivity (unlike the hemagglutination assay) and its fairly early detection (unlike the immunofluorescence assay).[33]
In a natural setting, the respiratory infection of Sendai virus in mice is acute. From the extrapolation of the infection of laboratory mice, the presence of the virus may first be detected in the lungs 48 to 72 hours following exposure. As the virus replicates in the respiratory tract of an infected mouse, the concentration of the virus grows most quickly during the third day of infection. After that, the growth of the virus is slower but consistent. Typically, the peak concentration of the virus is on the sixth or seventh day, and rapid decline follows that by the ninth day. A fairly vigorous immune response mounted against the virus is the cause of this decline. The longest period of detected presence of the virus in a mouse lung is fourteen days after infection.[34]
Eaton et al. advises that, when controlling an outbreak of SeV, disinfecting the laboratory environment and vaccinating the breeders, as well as eliminating infected animals and screening incoming animals, should clear the problem very quickly. Imported animals should be vaccinated with SeV and placed in quarantine, while, in the laboratory environment, breeding programs should be discontinued, and the non-breeding adults isolated for two months.[35]
Host restriction and safety for humans and domestic animals
Currently, there is no scientific data obtained using modern detection methods that would identify SeV as an infectious - disease causing agent for humans. Modern methods for the identification of pathogenic microorganisms have never detected SeV in pigs or other domestic animals, despite the isolation of other paramyxoviruses.[36][37][38][39][40][41] Consequently, it is recognized that Sendai virus disease causing infection is host restrictive for rodents and the virus does not cause disease in humans[42] or domestic animals, which are natural hosts for their own parainfluenza viruses.
Historic safety concerns
In 1952, Kuroya and his colleagues attempted to identify an infectious agent in human tissue samples at Tohoku University Hospital, Sendai, Japan. The samples were taken from the lung of a newborn child that was affected by fatal pneumonia. The primary isolate from the samples was passaged in mice and subsequently in embryonated eggs.[43][44] The isolated infectious agent was later called Sendai virus, which was used interchangeably with the name “Hemagglutinating Virus of Japan”. Kuroya and his colleagues were convinced that they isolated the virus, which is a new etiological agent for human respiratory infections. Later in 1954, Fukumi and his colleagues at the Japan National Institute of Health put forward an alternative explanation for the origin of the virus. It was suggested that the mice used to passage the virus were infected with the mouse virus. Thus, mouse virus was later transferred to embryonated eggs, isolated and finally named the Sendai virus.[45] This explanation of Fukumi, pointing to the mouse rather than the human origin of the virus, has been supported by numerous scientific data later. The historical aspects of the Sendai virus isolation and controversy behind it are well described in the review.[1] Thus, for some time, it was erroneously assumed that Sendai virus is human disease causing pathogen.[46] The incorrect assumption that the virus was isolated from human infectious material is still reported by Encyclopedia Britannica[47] and by ATCC in the description of the history of the viral isolate Sendai/52. It was also believed that the virus could cause disease not only in humans but also in pigs, because antibodies to the virus were often found in their organisms during the swine epidemic in Japan in 1953-1956. High incidence of seropositivity to the virus was observed in pigs in 15 districts of Japan.[46] An explanation was later found for this widespread detection of antibodies (see the section below). Yet, despite overwhelming evidence that indicate that SeV is host restrictive rodent pathogen, in some veterinary manuals and safety leaflets, SeV is still listed as a virus that can cause disease in pigs. Similar information is provided by Encyclopedia Britannica.[47] In reality, the multiple isolates of paramyxoviruses in pigs, using modern nucleic acid sequencing methods, have never been identified as SeV.[36][37][38][39][40][41]
Antigenic stability and cross-reactive antibodies
All viruses in the Paramyxoviridae family are antigenically stable, therefore porcine parainfluenza 1,[38][39] which is a close relative of SeV, most likely, has cross-reactive antibodies with SeV. This cross-reactivity may explain why SeV antibodies were found in pigs, and why it was thought that SeV was the etiological causative agent of pigs disease in Japan in 1953-1956.[46] Human parainfluenza virus type 1, also shares common antigenic determinants with SeV and triggers the generation of cross-reactive neutralizing antibodies.[48] This fact can explain wide spread detection of SeV antibodies in humans in the 1950-1960s.[46] After experimental SeV infection the virus can replicate and shed from the upper and lower respiratory tract of African green monkeys and chimpanzees, but it is not causing any disease.[49]
Interferon-related SeV replication restriction mechanism
SeV can overcome antiviral mechanisms in some of its natural hosts (rodents), but the virus is ineffective in overcoming these mechanisms in some virus resistant rodents and in other organisms that are not natural hosts of the virus such as humans.[50] Normal cells respond to viral infections by activating specific defense mechanisms. Virus-induced cell death due to apoptosis, which limits the spread of infection, is one such mechanism. In addition to stimulating the production of IFN, SeV triggers the production of other cytokines that provide protection against viruses (see "Stimulation of the secretion of interferons (IFN) type I and II" and "Stimulation of the secretion of immunostimulating antitumor chemokines and cytokines" sections below).
SeV activates the interferon (IFN) production pathway.
Activation of NF-kB, IRF-3 and IRF-7
SeV can activate the ubiquitously expressed interferon regulatory factor 3 (IRF-3), by triggering its post-translational phosphorylation in human cells. IRF-3, is activated by phosphorylation on a specific serine residue, Ser396.[51] There is also some evidence that demonstrates that SeV activates not only IRF-3, but the transcription factors NF-κB[52] and also IRF-7.[53] This stimulates IFN production and IFN signal transduction pathway that finally should lead to cell apoptosis.[54]
Activation of MAPK/ERK pathway
SeV replication triggers activation of MAPK/ERK pathway (also known as the Ras-Raf-MEK-ERK pathway) in a RIG-I-dependent manner in dendritic cells (DC) and in fibroblasts. RIG-I-mediated activation of this pathway by SeV results in type I IFN production.[55]
Activation of pattern recognition receptors (PRR)
RIG-1
IRF7-mediated induction of IFN-α by SeV requires both RIG-I and mitochondrial antiviral-signaling protein (MAVS) expression.[56] MAVS is also needed for SeV induction of IκB kinase (IKK), IRF3 and IFN-β in human cells.[57]
MDA5
Melanoma differentiation-associated antigen 5 (MDA5) has been shown to be an important participant in the antiviral SeV response and IFN type I production.[58]
IFN production by dendritic cells
Monocytes[59] and dendritic cells[60] produce IFN alpha/beta in response to SeV stimulation. However, plasmacytoid dendritic cells (pDC) ,dispite inability to be infected by SeV,[61] produce higher level of IFN-1 compared to monocytes and monocyte-derived dendritic cells in response to SeV. This happens most likely due to the higher levels of constitutively expressed IRF-7 in pDC compared to monocytes and monocyte-derived dendritic cells.[62]
The recognition of SeV by pDC happens through TLR7 activation and requires transport of cytosolic viral replication products into the lysosome by the process of autophagy. Moreover, for pDC, autophagy was found to be required for these cells production of IFN-α.[61]
Among conventional DCs only two subsets: CD4+ and CD8α−CD4− “double negative”[63] dendritic cells produce IFN-α and IFN-β in response to SeV infection. However, all conventional DC subsets including CD8α+ can be infected with SeV.[64] SeV can replicate to high titers in human monocyte-derived DCs.[65] However, pDCs are not producing a significant number of SeV virions after infection.[61] UV-irradiated SeV triggers lower levels of IFN-α production in pDC compared to alive virus.[61]
IFN production by mast cells
Human mast cell infection SeV induces the expression of type 1 IFN.[66]
Interferon response pathway helps protect against SeV infection
SeV can stimulate and/or inhibit the IFN-beta response pathway depending on the type of cell and host. Multiple examples of IFN-beta protecting cells from SeV infection are described It has been shown that SeV infects human lung fibroblasts MRC-5 and induces the release of IFN-beta into the culture medium from infected cells. Pretreatment of these cells with IFN-beta inhibits the replication of SeV.[50]
A similar IFN-beta protection against the virus has been observed for some human malignant cells that maintain the IFN response pathway. HeLa cells can be infected with SeV, however, incubation of these cells with IFN-beta causes inhibition of SeV replication.[67] Multiple interferon stimulated genes (ISG) were identified as being required for this inhibition including IRF-9, ZNFX1, TRIM69, NPIP, TDRD7, PNPT1 and so on.[67] One of this genes TDRD7 was investigated in more detail. The functional TDRD7 protein inhibits the replication of SeV and other paramyxoviruses, suppressing autophagy, which is necessary for productive infection with these viruses.[67]
IFN also triggers the expression of IFN induced Ifit2 protein that is involved in protecting mice from SeV through an as yet unknown mechanism.[68]
Stimulation of inflammasome helps protect against SeV infection
Using human embryonic kidney cells (HEK 293T) it has been shown that SeV can stimulate production of a pattern recognition receptor NLRC5, which is a cytosolic protein expressed mainly in hematopoetic cells.[69] This production activates the cryopyrin (NALP3) inflammasome.[70] Using human monocytic cell line-1 (THP-1) it has been shown that SeV can activate signal transduction by mitochondrial antiviral-signaling protein signaling (MAVS), which is a mitochondria-associated adaptor molecule that is required for optimal NALP3-inflammasome activity. Through MAVS signaling SeV stimulates the oligomerization of NALP3 and triggers NALP3-dependent activation of caspase-1[71] that, in turn, stimulates caspase 1-dependent production of inteleukine -1 beta (IL-1β).[72]
Stimulation of beta-defensins
SeV is a very effective stimulant of expression of human beta-defensin-1 (hBD-1). This protein is a member of the beta-defensin family of proteins that bridges innate and adaptive immune responses to a pathogen infection.[73] In response to SeV infection, the production of hBD-1 mRNA and protein increases 2 hours after exposure to the virus in purified plasmacytoid dendritic cells or in PBMC.[74]
SeV activates strong anti-viral response in human mast cells
Human mast cell infection with SeV induces an antiviral response with activation of expression of type 1 IFN, melanoma differentiation–associated gene 5 (MDA-5), retinoic acid-inducible gene-1 (RIG-1), and toll-like receptor 3 (TLR-3). In addition, SeV infection of mast cells induces the anti-viral proteins MxA and IFIT3.[66]
As an oncolytic agent
Sendai virus-based anticancer therapy for model[2][3] and companion animals[4] has been reported in several scientific papers. The described studies demonstrate that Sendai virus has a potential of becoming a safe and effective therapeutic agent against a wide range of human cancers.
Safety for humans
One of the great advantages of the Sendai virus as a potential oncolytic agent is its safety. Even though the virus is widespread in rodent colonies[1] and has been used in laboratory research for decades,[75] it has never been observed that it can cause human disease. Moreover, Sendai virus has been used in clinical trials involving both adults[48] and children[76] to immunize against human parainfluenza virus type 1, since the two viruses share common antigenic determinants and trigger the generation of cross-reactive neutralizing antibodies.The Sendai virus administration in the form of nasal drops in doses ranging from 5 × 105 50% egg infectious dose (EID50) to 5 × 107 EID50 induced the production of neutralizing antibodies to the human virus without any measurable side effects.The results of these trials represent additional evidence of Sendai virus safety for humans.
Model cancers
For cancer studies, it is desirable that the oncolytic virus be non-pathogenic for experimental animals, but the Sendai virus can cause rodent disease, which is a problem for research strategies. Two approaches have been used to overcome this problem and make Sendai virus non-pathogenic for mice and rats. One of these approaches included the creation of a set of genetically modified attenuated viral strains. Representatives of this set were tested on model animals carrying a wide range of transplantable human tumors. It has been shown that they can cause suppression or even eradication of fibrosarcoma,[77][78] neuroblastoma,[79] hepatocellular carcinoma,[80] melanoma, squamous cell and prostate carcinomas.[81] Complete eradication of established gliosarcomas in immunocompetent rats has also been observed.[82] SeV constructs have also been created with a modified protease cleavage site in the F-protein. The modification allowed the recombinant virus to specifically infect cancer cells that expressed the corresponding proteases.[80]
Another approach of making Sendai virus non-pathogenic included the short-term treatment of the virions with ultraviolet light. Such treatment causes a loss of the virus replication ability. However, even this replication-deficient virus can induce the cancer cells death and stimulate anti-tumor immunity. It can trigger extensive apoptosis of human glioblastoma cells in culture, and it can efficiently suppress the growth of these cells in model animals.[83] The ultraviolet light treated virus can also kill human prostate cancer cells in culture[84] by triggering their apoptosis and eradicate tumors that originated from these cells in immunodeficient model animals.[85] Moreover, it can stimulate immunomodulated tumor regression of colon[86] and kidney cancers[87][88] in immunocompetent mice. Similar regressions caused by the replication-deficient Sendai virus have been observed in animals with transplanted melanoma tumors.[89][90]
Natural cancers
Some cancer studies with non-rodent animals have been performed with the unmodified Sendai virus. Thus, after intratumoral injections of the virus, complete or partial remission of mast cell tumors (mastocytomas) was observed in dogs affected by this disease.[4] Short-term remission after an intravenous injection of SeV was described in a patient with acute leukemia treated in the Clinical Research Center of University Hospitals of Cleveland (USA) by multiple viruses in 1964.[91] It is also reported[3][92] that the Moscow strain of SeV[93] was tested by Dr. V. Senin[94] and his team as an anticancer agent in a few dozen patients affected by various malignancies with metastatic growth in Russia in the 1990s.[95] The virus was injected intradermally or intratumorally and it caused fever in less than half of the treated patients, which usually disappeared within 24 hours. Occasionally, the virus administration caused inflammation of the primary tumor and metastases. Clinical outcomes were variable. A small proportion of treated patients experienced pronounced long-term remission with the disappearance of primary tumors and metastases. Sometimes the remission lasted 5–10 years or more after virotherapy. Brief descriptions of the medical records of the patients that experiences long-term remission are presented in the patent.[95]
Anticancer Mechanism
Direct cancer cells killing. Malignant cells are vulnerable to SeV infection.
Sendai virus receptors are often overexpressed in cancer cells.
Some cell entry receptors for SeV (see the “Cell receptors” section below) are overexpressed in cancer cells, and their expression is important for carcinogenesis and/or metastasis. For example, the presence of Sialyl-Lewisx antigen on the outer cell membrane correlates with invasion of malignant cells, tumor recurrence, and overall patient survival for an extremely wide range of cancers.[96] Expression of the Vim2 antigen is very important for the extravascular infiltration process of acute myeloid leukemia cells.[97] GD1a,[98] ganglioside is found in large quantities on the surfaces of breast cancer stem cells.[99] High cell surface expression of another ganglioside sialosylparagloboside /SPG/ NeuAcα2-3PG.[100] characterizes lymphoid leukemia cells.[101][102] GT1b, ganglioside is highly expressed on the outer membranes of brain metastases cells that originate from an extremely broad range of cancers.[103]
Sendai virus proteolytic processing enzymes are often overexpressed in cancer cells.
The fusion protein (F) of SeV is synthesized as an inactive precursor and is activated by proteolytic cleavage of the host cell serine proteases (see the section “Proteolytic cleavage by cellular proteases” below). Some of these proteases are overexpressed in malignant neoplasms. For example, transmembrane serine protease 2 (TMPRSS2), which is an F-protein-processing enzyme, is often overexpressed in prostate cancer cells. It is also overexpressed in some cell lines originating from various malignant neoplasms. Thus, it is highly expressed in bladder carcinoma RT4 urinary bladder carcinoma RT4, human colon carcinoma CaCo2[104] and breast carcinomas SK-BR-3, MCF7 and T-47d.[105] Another F-protein-protease is tryptase beta 2 (TPSB2). This protease (with alias such as tryptase-Clara and mast cell tryptase) is expressed in normal club cells, mast cells and in some cancers.[106] It’s especially high expression is observed in the human mast cell line HMC-1,[107][108] and in the human erythroleukemia cell line HEL.[109][107] Plasminogen (PLG), from which originates the mini-plasmin that can cleave the F-protein, is highly expressed in liver cancers.[110] Its expression is also increased in a wide range of other malignant neoplasms.[110] Factor X (F10) is frequently expressed in normal liver and in liver cancers.[111] SeV constructs were created with a modified protease cleavage site. The modification allowed the recombinant virus to specifically infect cancer cells that expressed the corresponding proteases, which can cleave a modified protease cleavage site.[112][77][80]
Defects in the interferon system that characterize malignant cells promote oncolytic virus infection.
The interferon production and / or response system often malfunctions in malignant cells; therefore, they are much more vulnerable to infection with oncolytic viruses compared to normal cells.[113] Thus, cells belonging to three human cell lines, originated from variable malignancies, such as U937, Namalwa, and A549, retain their ability to become infected with SeV even after treatment with type 1 IFN. Broken interferon response system cannot protect these cells from SeV infection.[114]
The Namalwa cells, although there was some defect in the system of response to interferon, has been shown that SeV virus stimulates an expression of many genes involved in immune defense pathways, such as type I and type II IFN signaling, as well as cytokine signaling. Among the ten most virus-induced mRNAs are IFNα8, IFNα13, IFNβ, IFNλ:(L28α, IL28β, IL29), OASL, CXCL10, CXCL11 and HERC5.[115]
Virus, mediated fusion of cancer cells, kills them faster.[3]
The host organism fights viral infection using various strategies. One such strategy is the production of neutralizing antibodies. In response to this production, viruses have developed their own strategies for spreading the infection and avoiding the inactivation by the host produced neutralizing antibodies. Some viruses, and in particular paramyxoviruses, can produce new virus particles by fusing infected and healthy host cells. This fusion leads to the formation of a large multi-nuclear structure (syncytium). Sendai virus, as a representative of Paramyxoviridae, uses this strategy to spread its infection (see the section “Directed cell fusion” below). The virus can fuse up to 50-100 cells adjacent to one primary infected cell. This multi-nuclear formation, derived from several dozens of cells, survives for several days and subsequently releases functional viral particles.
It has been demonstrated that the ability of a virus to destroy tumor cells increases along with an increase in the ability of the virus to form large multi-nuclear structures. The transfer of genes that are responsible for the formation of syncytium from the representative of Paramyxoviridae to the representatives of Rhabdoviridae or Herpesviridae makes the recipient viruses more oncolytic.[116][117] Moreover, the oncolytic potential of paramyxovirus can be enhanced by mutations in the fusion (F) gene protease-cleavage site, which allows the F-protein to be more efficiently processed by cellular proteases.[118] The introduction of the F gene of SeV in the form of a plasmid into the tumor tissue in mice by electroporation showed that the expression of the F gene increases the T cell infiltration of the tumor with CD4 + and CD8 + cells and inhibits tumor growth.[119] It was also shown in other similar experiments that cancer cells themselves, transfected with plasmids that encode viral membrane glycoproteins with fusion function, cause the collective death of neighboring cells forming syncytium with them. Recruitment of bystander cells into the syncytium leads to significant regression of the tumor.[120][121][122]
Immunomodulated cancer cells killing. Destruction of malignant cells by specific anti-tumor immunity triggered by the virus
The virus triggers indirect immunomodulated death of malignant cells using a number of mechanisms, which are described in a published review.[3] The viral enzyme neuraminidase (NA), which has sialidase activity, can make cancer cells more visible to the immune system by removing sialic acid residues from the surface of malignant cells.[3] SeV activates natural killer cells (NK), cytotoxic T lymphocytes (CTL) and dendritic cells (DC). The secretion of interleukin-6, that is triggered by the virus, also inhibits regulatory T cells.
Stimulation of the secretion of cytokines
Interferons (IFN)
Type 1 and type II interferons have anticancer activity (see the "Function" section in the "Interferon" article). Sendai-virus-induced human peripheral blood leukocytes produce the interferon alpha (IFN-α)[123] and the interferon gamma (IFN-γ).[124][125] Interferon-beta (IFN-β) production in human fibroblast cells also occurs in response to SeV treatment.[126] Because of powerful IFN stimulating properties, before recombinant IFN became available for medical use, SeV was selected, among other viruses, for the industrial large-scale IFN production. A procedure involving inactivated SeV treatment of human peripheral blood leukocytes from donors’ blood was used for this production.[127] The SeV induced IFN-α consists from at least nine different sub types of IFN-α: 1a, 2b, 4b, 7a, 8b, 10a, 14c, 17b and 21b. Among these sub types IFN-α1 represents about 30% of total IFN-α.[128]
The Namalwa cells, which originated from human Burkitt lymphoma, has been shown that SeV virus stimulates an expression of IFNα8, IFNα13, IFNβ and IFN type III (IFN-lambda, IFNλ):(L28α, IL28β, IL29).[115]
UV-inactivated SeV can induce the production of type I IFN by some mouse dendritic cells,[129] and by some tumor cell lines.[85] However, UV-inactivated SeV triggers lower levels of IFN-α production in pDC compared to alive virus.[61] Most likely the glycoprotein HN is responsible for the effect of triggering type I IFN. It was shown that the HN of Paramyxoviruses is a potent inducer of type 1 IFN in human blood mononuclear cells[130] and the HN of SeV can induce type 1 IFN production in mouse spleen.[131]
It has been demonstrated that SeV can induce the production of IFN type III (IFN-lambda)[132] by human plasmacytoid dendritic cells.[133]
Non interferons
Sendai virus can induce the production of many cytokines that enhance cellular immune responses against cancer cells. SeV can stimulate the production of macrophage inflammatory protein-1α (MIB-1α) and –β (MIB-1β), RANTES (CCL5), tumor necrosis factor-alpha (TNF-alpha), tumor necrosis factor-beta (TNF-beta), interleukin-6 (IL-6 ), interleukin-8 (IL-8), interleukin-1 alpha (IL1A), interleukin-1 beta (IL1B), platelet-derived growth factor (PDGF-AB) and small concentrations of interleukin-2 (IL2) and GM-CSF.[125][134][135] Even plasmids that deliver the F-coding gene of SeV to tumor cells in model animals trigger the production of RANTES (CCL5) in tumor-infiltrated T-lymphocytes.[136]
SeV induces the production of B cell-activating factor by monocytes and by some other cells.[137]
Heat-inactivated SeV virus induces the production of IL-10 and IL-6 cytokines by dendritic cells (DC).[138] Most likely, F protein is responsible for this induction because reconstituted liposomes containing F protein can stimulate IL-6 production by DC. The production of IL-6 in response to SeV infection is restricted to conventional dendritic cells (DCs) subsets, such as CD4+ and double negative (dnDC).[64]
The UV-inactivated SeV (and likely the alive virus as well) can stimulate dendritic cells to secrete chemokines and cytokines such as interleukin-6, interferon-beta, chemokine (C-C motif) ligand 5, and chemokine (C-X-C motif) ligand 10. These molecules activate both CD8+ T cells as well as natural killer cells and attract them to the tumor. It has been shown that in cancer cell lines, UV-inactivated SeV triggers the production of an intercellular adhesion molecule -1 (ICAM-1, CD54), which is a glycoprotein that serves as a ligand for macrophage-1 antigen (Mac-1) and lymphocyte function-associated antigen 1 (LFA-1 (integrin)). Mac-1 and LFA-1 are receptors found on leukocytes. This induced production happens through the activation of nuclear factor-κB downstream of the mitochondrial antiviral signaling pathway and the retinoic acid-inducible gene I. The increased concentration of ICAM-1 on the surface of cancer cells, which is triggered by SeV, increases the vulnerability of these cells to natural killer cells.[139]
Neuraminidase (NA) removal of sialic acid from the surface of malignant cells stimulates natural killers cells and cytotoxic T lymphocytes
Elevated levels of cell membrane sialylation are associated with increased cancer cell potential for invasion and metastasis and with progression of the malignancy.[140][141][142][143][144][145] Some sialylation inhibitors can make cancer cells less malignant.[146][147][148]
One possible explanation for the relationship between increased sialylation and a malignant phenotype is that sialylation results in a thick layer of coating on the cell membrane that masks cancer antigens and protects malignant cells from immune surveillance. The activity and cytotoxicity of NK cells is inhibited by the expression of sialic acids on the tumor cell surface.[149] Removal of sialic acid residues from the surface of tumor cells makes them available to NK cells and cytotoxic T lymphocytes and, therefore, reduces their growth potential. Moreover, treating tumor cells with sialidase improves activation of NK cell secretion of IFN-γ.[149]
Some paramyxoviruses, including SeV encode and synthesize neuraminidase (sialidase), which can remove sialic acid residues from the surface of malignant cells. Hemagglutinin-neuraminidase (HN) is a single protein that induces hemagglutination and possesses neuraminidase (sialidase) activity. Neuraminidase (NA), a subunit of the HN protein, binds to and cleaves sialic acid from the cell surface.[150] NA also promotes cell fusion, which helps the nascent virions to avoid contact with host antibodies and thus enables the virus to spread within tissues.
Sialidase treatment of cells causes loss of sialic acid residues. This loss significantly increases the ability of malignant cells to activate cytotoxic T lymphocytes.[151] Variable sialidases can cause this effect,[151] including NA from Newcastle disease virus that have been shown to cleave 2,3-, 2,6-,[152] and 2,8-linkages between sialic acid residues.[153] In vitro, there was no significant difference between NAs from Newcastle disease virus, SeV and mumps virus[154] with respect to substrate specificity. These results suggest that treating a tumor with the virus results in desialylation of malignant cells, which contributes to increased anti-tumor immune surveillance. Therefore, the ability of SeV sialidase (NA) to remove sialic acid from the surface of malignant cells most likely helps to ensure the availability of tumor antigens for recognition by cytotoxic T lymphocytes.
SeV stimulation of natural killer (NK) cells
Experiments with UV-inactivated SeV showed that NK cells are important in virus-mediated inhibition of tumor growth. This was shown in a mouse model of renal cancer, in which the anti-tumor effect of SeV was suppressed by reducing the number of NK cells by co-injection of specific antibodies.[155]
The activation of NK requires several receptors, among which are natural killer proteins 46 (NKp46) and 44 (NKp44). Studies have shown that the only paramyxovirus protein that activates NK is HN.[156] HN protein binding to NKp46 and/or NKp44 results in the lysis of cells whose surfaces display the HN protein or its fragments.[157][158] It can be assumed that NK activation and tumor suppression by UV-treated SeV[155] are caused by interaction between HN belonging to SeV, and NKp46 and/or NKp44 receptors belonging to NK cells.
Paramyxovirus induction of anti-tumor cytotoxicity of CTL
SeV even after UV inactivation can cause tumor infiltration by dendritic cells (DCs) and CD4+ and CD8+ T and it also can cause enhancing of anti-tumor activity of these cells.[86] Most likely, viral hemagglutinin-neuraminidase protein, highly contributes to the effect (see "Neuraminidase (NA) removal of sialic acid from the surface of malignant cells stimulates natural killers cells and cytotoxic T lymphocytes" section above).This hypothesis is based on two observations. First, the functional hemagglutinin-neuraminidase protein of the oncolytic Newcastle disease virus (NDV), which is a relative of SeV, has been shown to enhance the tumor-specific cytotoxic response of CD8+ T-cells and to increase the activity of CD4+ T-helper cells.[158] Second, UV-inactivated NDV, which is unable to replicate, promotes anti-tumor CTL response as well as does intact NDV which is able to replicate.[158] Since the hemagglutinin-neuraminidase proteins of the SeV and NDV viruses are highly homologous, it is likely that the HN protein of the SeV virus can activate both CTL and natural killers cell responses. Most likely neuraminidase removal of sialic acid from the surface of malignant cells contributes to this effects.
SeV stimulation of dendritic cells
UV-inactivated SeV can cause dendritic cells (DCs) to maturate and to infiltrate a tumor,[86] and ex vivo infection of DCs with recombinant SeV induces maturation and activation of DCs within 60 minutes.[159] When activated DCs that carry variants of recombinant SeV are administered, survival of animals injected with malignant melanoma,[160][161] colorectal cancer,[162] squamous cell carcinoma,[163] hepatic cancer, neuroblastoma, and prostate cancer[164] is significantly improved. It has been shown that the administration of such DCs prior to tumor cell injection prevents metastasis of neuroblastoma and prostate adenocarcinoma to the lungs.[165][166]
SeV can replicate to high titers in human monocyte-derived DCs.[65] With the multiplicity of infection of 2, approximately 1/3 of the DCs begin to express encoded SeV proteins 8 hours after infection. This proportion increases to 2/3, 24 hours and decreases to 1/3, 48 hours after infection. SeV demonstrates high cytopathic effect on DCs; the virus can kill a third of DC even with a very low multiplicity of infection such as 0.5. Most important observation is that SeV infection triggers DC maturation, which is manifested in DC cell surface markers composition. The virus increases the expression of class I and class II molecules of the major histocompatibility complex (MHC) (HLA-A, HLA-B, HLA-C and HLADR), CD83, as well as costimulatory molecules CD40 and CD86.[167]
SeV suppression of regulatory T cells
Experiments with animal models have shown that, even after UV inactivation, SeV can block T-cell-mediated regulatory immunosuppression in tumors. The blocking mechanism is associated with the stimulation of SeV inactivated virions of interleukin 6 (IL-6) secretion by mature DCs. These effects lead to the eradication of most model tumors and inhibit the growth of the rest.[86] It has been shown that F protein alone can trigger IL-6 production in DC in a fusion-independent manner.[136]
As a vector[168]
SeV has been known to the research community for over 60 years. Over the past three decades, it has been widely used to create a wide range of genetic engineering constructs, including vectors for trans-genes delivery.[169] Due to exclusively cytoplasmic replication, the virus does not carry the risk of genetic integration into the host genomes, which is a problem for many other viral vectors.
Genes addition, deletion and modification
Recombinant SeV variants has been constructed by introducing new genes and/or by deleting some viral genes such as F, M, and HN from the SeV genome,[170][171][172] SeV constructs have also been created with a modified protease cleavage site.[112][77][80]
Non-invasive imaging
A set of different recombinant SeV constructs was created for non-invasive imaging of the virus infection in animals. The constructs allow to study dynamics of SeV spread and clearance. One construct, rSeV-GFP4, which is commercially available, can deliver a green fluorescent protein (GFP4) to a cell. Another one rSeV-luc(M-F*) – luciferase.[173][174]
Reprogramming into induced pluripotent stem cells (iPSCs)
One of the latest applications of SeV-based vectors is the reprogramming of somatic cells into induced pluripotent stem cells.[175][176][177][112] The resulting iPSCs became trans-gene free.[178] The system for such reprogramming is commercially available from ThermoFisher Scientific as CTS™ CytoTune™-iPS 2.1 Sendai Reprogramming Kit, Catalog number: A34546.[179]
Virus biology and properties
Virion structure
Virion structure is well described in a published review.[1] Sendai virus is an enveloped virus: its outer layer is a lipid envelope, which contains glycoprotein hemagglutinin-neurominidase (HN)[180] with two enzymatic activities (hemagglutinating and neuraminidase).[181] Hemagglutinin (H) serves as a cell attachment factor and membrane fusion protein. Neuraminidase (NA) is a sialidase that cleaves and removes sialic acid from the surface of a host cell. This cleavage promotes the fusion of the viral lipid envelope with the cell outer membrane.
In the lipid envelope of the virus located a fusion protein (F),[182] which is also a glycoprotein that ensures the virus entry into a host cell after viral adsorption. Under the lipid membrane is a matrix protein (M);[183] it forms the inner layer of the virus envelope and stabilizes it structure. The SeV virion also contains the nucleocapsid core, which is composed of the genomic RNA, the nucleocapsid protein (NP),[184] the phosphoproteins (P)[185] and the large protein (L)[186] that is the catalytic subunit of RNA-dependent RNA polymerase (RDRP). C-protein, which is translated from an alternative reading frame of the P-coding mRNA, is also associated with a viral capsid.[187]
Proteolytic cleavage by cellular proteases
The SeV F protein is a type I membrane glycoprotein that is synthesised as an inactive precursor (F0) that must be activated by proteolytic cleavage at residue arginine-116.[188] After the cleavage F0 precursor yields two disulfide-linked subunits F1 and F2.[189] Paramyxoviruses use different host cell proteases to activate their F-proteins. Sendai virus uses activating proteases, which are serine endopeptidases represented by tryptase beta 2-(TPSB2), which has aliases such as tryptase II, tryptase Clara, club cells tryptase, mast cells tryptase,[190][191][192][193] trypsin 1 (PRSS1),[194] mini-plasmin (PLG)[195] and transmembrane serine protease 2 (TMPRSS2).[196] Most likely, blood clotting factor X (F10) is capable to cleave and activate SeV F0.[197][198][199] It is possible that other, not yet identified cellular proteases, can also process the F0 protein of SeV.
Genomic structure
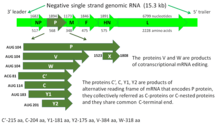
The SeV genome is non-segmented, negative-sense RNA, of about 15.384 n. in length, and contains the noncoding 3’ leader and 5’ trailer regions, which are about 50 nucleotides in length.[188][200] As in other respiroviruses from Paramyxoviridae family, in SeV they work as cis-acting elements essential for replication. A 3’ leader sequence acts as a transcriptional promoter. Between these non-coding regions are located six genes, which encode the nucleocapsid (NP) protein, phosphoprotein (P), matrix protein (M), fusion protein (F), hemagglutinin-neuraminidase (HN) and large (L) protein in this order from the 3’ terminus.[188][200] Intergenomic regions between these genes are three nucleotides long as in other respiroviruses. Additional proteins, which are frequently called non structural or accessory proteins can be produced from the P gene, using alternative reading frames.[188][201] The Sendai virus P/C mRNA contains five ribosomal initiation sites between positions 81 and 201 from the 5' end. One of these sites initiates in the P open reading frame, whereas four others initiate a nested set of C proteins (C', C, Y1, Y2).[202][201][203] These C proteins are initiated in the + 1 reading frame to that of P at different translation starting sites. Sendai virus uses ribosome shunting to express Y1 and Y2 proteins that initiate at the fourth and fifth start sites on the P/C mRNA (respectively).[203] Three additional SeV proteins are also encoded by P/C mRNA. Two of these proteins V and W are products of RNA editing, at codon 317 of the mRNA - G residues are added co-transcriptionally, (+one G residue for V and +two G for W).[204] The third - X protein is represented by 95 amino acids of the C terminal of the P protein and independently initiated by ribosomes.[205] All these non-structural proteins have several functions, including the organization of viral RNA synthesis and helping the virus to infect rodent cells by escaping host innate immunity (see "The mechanism of viral immunosuppression in natural hosts" section above).[204] It has also been found that C protein facilitates budding of virus-like particles[206] and small amounts of C protein are associated with a viral capsid.[187]
SeV cell receptors identity
The strains of respiroviruses, avulaviruses, and the majority of rubulaviruses, that have HN in their envelopes, use sialic acids as their cell entry receptors. SeV, as a representative of respiriroviruses, uses lectin and the molecules containing sialic acids residues.
Three SeV receptors are represented by molecules that are clusters of differentiation.[207]
Type and sub-type of receptor-molecule | Receptor | Affinity to SeV |
LECTINS | ||
C-type lectin | Asialoglycoprotein receptor (ASGP-R)[208] | Not reported |
GLYCOPROTEINS | ||
Bovine glycoprotein 2 | Glycoprotein 2/ GP2[209] | Not reported |
Human sialoglycoprotein - cluster of differentiation | Glycophorin A/ GYPA/ CD235a[210] | High |
FUCOSYLATED GLYCANS (can be attached to lipids or proteins) | ||
Tetrasaccharide - cluster of differentiation | Sialyl-Lewis x antigen/ sLeX/CD15s[211] | High |
Ceramide-dodecasaccharide - cluster of differentiation | Vim2 antigen/CD65s/α2,3-sialylatedceramidedodecasaccharide 4c[212][211] | |
GANGLIOSIDS | ||
Ganglio-series[213][214][215][216][217] | a-series GM3 | Low |
a-series GD1a,[98] b-series GT1b[218] | Moderate | |
a-series GT1a,[219] c-series GP1c[220] | High | |
b-series GQ1b[221] | Very high | |
Neolacto-series[216][222][217] | NeuGca2-3I, Sialoparagloboside/NeuAca2-6PG, NeuAca2-6I[223] | Moderate |
NeuAcα2-3I, NeuAcα2-3i, Sialosylparagloboside /SPG/ NeuAcα2-3PG[100] | Very high
|
The structures of some of these receptors are available for visualization through SugarBindDB - a resource of glycan-mediated host–pathogen interactions.[224] Others are available through KEGG Glycan Database,[225] PubChem compound database,[226] and TOXNET database (toxicology data network) of US National Library of Medicine.[227]
SeV receptors are potential biomarkers for assessing the susceptibility of cancer cells to the virus
Since SeV receptors are potential biomarkers for evaluation of the vulnerability of malignant cells to the virus, it is important to measure their expression level. Cellular expression of glycoproteins can be evaluated by various methods, which include RNA and protein measurements. However, cellular expression of gangliosides, which are sialic acid-containing glycosphingolipids, can not be evaluated by these methods. Instead it can be measured using anti-glycan antibodies, and despite the large collection of such antibodies in a community resource database, they are not always available for each ganglioside.[228] Therefore, indirect measurement of ganglioside expression by quantifying the levels of fucosyltransferases and glycosyltransferases that complete glycan synthesis is an alternative.There is evidence that expression of these enzymes and the production of gangliosides strongly correlate.[102]
Processing transferases for SeV receptors
At least four representatives of fucosyltransferases and several glycosyltransferases including sialyltransferases are responsible for the synthesis of gangliosides that can serve as SeV receptors. All of these proteins are often overexpressed in various tumors, and their expression levels correlate with the metastatic status of the tumor and the shorter life span of the patients. Thus, these enzymes are also potential biomarkers of SeV-oncolytic infectivity
SeV receptor | Type of enzyme | Enzyme |
Sialyl-Lewisx antigen/(sLeX/CD11s)[229][230][231][232] | Fucosyltransferase | FUT3, FUT5, FUT6, FUT7 |
Glycosyltransferase
(Sialyltransferase) |
ST3GAL3, ST3GAL4, ST3GAL6, | |
Vim2 antigen /(CD65)[231] | Fucosyltransferase | FUT5 |
GD1a[233][234][102][235] | Glycosyltransferases
(Sialyltransferase) |
ST3GAL1, ST3GAL2, ST6GALNAC5 ST6GALNAC6 |
GD1b,[234] (GT1a, GQ1b and GP1c)[233] | ST6GALNAC6 | |
GT1b[236] | ST3GAL2, | |
Sialosylparagloboside (SPG).[102] | ST3GAL6, |
Directed cells fusion (syncytium formation)
One recognized feature of the Sendai virus, shared with members of its genus, is the ability to induce syncytia formation in vivo and in vitro in eukaryotic cell cultures.[237] The formation of syncytium helps the virus to avoid neutralizing antibodies of the host organism during the spread of infection.The mechanism for this process is fairly well understood and is very similar to the fusion process employed by the virion to facilitate cellular entry. The activities of the receptor binding hemagglutinin-neuraminidase protein is solely responsible for inducing close interaction between the virus envelope and the cellular membrane.
However, it is the F protein (one of many membrane fusion proteins) that, when triggered by local dehydration[238] and a conformational change in the bound HN protein,[239] actively inserts into the cellular membrane, which causes the envelope and the membrane to merge, followed shortly by virion entry. When the HN and F protein are manufactured by the cell and expressed on the surface, the same process may occur between adjacent cells, causing extensive membrane fusion and resulting in the formation of a syncytium.[240]
This behavior of SeV was utilized by Köhler and Milstein, who published an article in 1975 outlining a revolutionary method of manufacturing monoclonal antibodies. In need of a reliable method to produce large quantities of a specific antibody, the two merged a monoclonal B cell, exposed to a chosen antigen, and a myeloma tumor cell to produce hybridomas, capable of being grown indefinitely and of producing significant amounts of an antibody specifically targeting the chosen antigen. Though more efficient methods of creating such hybrids have since been found, Köhler and Milstein first used Sendai virus to create their revolutionary cells.[5]
Sensitive cell lines
Scientific studies show that the following cell lines are susceptible to SeV infection to varying degrees.
Cell line | Origin |
CaCo2[196] | human colon carcinoma |
Hep G2[208][241][242] | human liver carcinoma |
Huh7[243][241] | human well differentiated hepatocyte-derived carcinoma |
MCF7[244] | human breast adenocarcinoma |
A549[245][242][114] | human lung carcinoma |
Calu-3[246] | human lung carcinoma |
HeLa,[242] | human cervical carcinoma |
U937[114] | human histiocytic lymphoma |
U87MG[247] | most likely, human glioma |
Mel8[247] | human melanoma |
Namalwa[248][114] | human Burkitt lymphoma |
PC-3[249] | human prostate carcinoma derived from metastatic site in bone |
DU145[249] | human prostate carcinoma derived from metastatic site in brain |
4T1[242] | mouse mammary gland metastatic adenocarcinoma |
WD-PBEC[250] | human primary bronchial epithelial cells |
HEK 293[247] | human embryonic kidney cells |
Vero cell[242] | monkey kidney epitelial cells |
LLC MK2[251] | resus monkey kidney cells |
4647[247] | green monkey kidney cells |
BHK 21[242] | humster baby kidney fibroblasts |
L929[67] | murine fibroblasts |
Some of these cells (for example, 4647 and HEK 293) do not express a protease that processes fusion protein F0 of Sendai Virus; therefore, they produce non-infectious virions.[247]
Type 1 IFN inhibits the SeV production in normal human respiratory cells,[50] but fails of doing it in human cells that originates from variable malignancies such as U937, Namalwa, and A549.[114]
Primary cell cultures obtained from tumors of the same type of cancer can have different sensitivity to SeV, and can also produce the virus in different quantaties. This effect has been demonstrated using primary cultures of prostate cancer cells. It has been shown that prostate cancer cells, which are sensitive to SeV, have lower level of constitutive expression of TLR 3 and TLR 7. Thus, an inverse correlation was observed between cells sensitivity to SeV infection and constitutive mRNA expression levels of TLR 3 and TLR 7.[249]
SeV variants adapted for growth in different cells have different properties. One study shows that the SeV variant adapted for growth in LLC-MK2 cells and the SeV variant adapted for growth in embryonated eggs differ by two amino acids in the HN protein. This difference results in different neuraminidase conformations around the receptor binding site and variations in neuraminidase activity between the two viral variants.[252] Another research study shows that SeV variants, adapted to grow in cell culture 4647 (African green monkey kidney cells) and in HEK 293 (human embryonic kidney cells) instead of embryonated chicken eggs, also acquire mutations in HN gene and both SeV variants lose their oncolytic activity.[247][253]
Strains
History
All Sendai virus strains belong to the same serotype. The origin of many strains of SeV was described in 1978.[46] Some additional strains such as Ohita[254] and Hamamatsu[255] were described later. Ohita and Hamanatsu strains were isolated from separate epidemics in laboratory mice.[256][257] According to the personal memory of Alisa G. Bukrinskaya, who has co-authored numerous publications related to SeV along with Prof. Viktor M. Zhdanov, starting in 1961,[258] the Moscow strain of SeV[93] was obtained by Prof. Viktor M. Zhdanov of the Ivanovsky Institute of Virology from Japan in the late 1950s or early 1960s,[258] It is reported[259] that the BB1 strain[260] derived from the Moscow virus strain.[93] The strain BB1 was given to the researches of Institute of Viral Disease Control and Prevention, Beijing, China by researches of Ivanovsky Institute of Virology, Moscow, Russia in 1960s.[259]
Virulence
A field SeV isolate, that is attenuated through egg-passages, is less virulent for mouse respiratory cells.[261] Therefore, the strains that were isolated from animals a few decades ago and went through multiple passages in eggs are less virulent for mice in comparison with the strains that are fresh field isolates.
Defective interfering genomes
Defective interfering (DI) genomes or defective viral genomes (DVGs) are replication defective viral RNA products generated during viral infections by many types of viruses, including SeV.[262][263][264] A single amino acid substitution in a nucleoprotein (NP) causes an increased production rate of DI genomes in the SeV Cantell strain, which is known for its particularly strong induction of interferon beta (IFN-β) during viral infection.[265] It has been shown that DI are responsible for this strong IFN-β induction.[266]
Strains origin and sequence ID
Strain name | Origin | Sequence ID |
Z (Sendai/52, or VR-105, or Fushimi) | derivative of murine isolate of 50s (Japan) | AB855655.1 |
Cantell (VR-907) | derivative of the same isolate as above | AB855654.1 |
Enders | derivative of the same isolate as above | * |
Nagoya | derivative of the same isolate as above | AB275417.1 |
Moscow | derivative of murine isolate of 50-60s (Japan or Russia) | KP717417.1 |
BB1 | derivative of the same murine isolate of 50-60s as above (Japan or Russia) | DQ219803.1 |
Ohita | murine isolate of 70-90s (Japan) | NC_001552.1 |
Hamamatsu | independent from Ohita, murine isolate of 70-90s (Japan) | AB039658 |
*The sequence of Enders strain is available from the US patent
Strains sequence similarity
Strain name | Z | Cantell | Enders | Nagoya | Moscow | BB1 | Ohita | Hamamatsu |
Sendai Virus | Megablast homology for SeV complete genome (%) | |||||||
Z | 100 | |||||||
Cantell | 99.3 | 100 | ||||||
Enders | 99.4 | 99.2 | 100 | |||||
Nagoya | 98.9 | 100 | ||||||
Moscow | 88.1 | 88.6 | 87.9 | 100 | ||||
BB1 | 88.1 | 99.9 | 100 | |||||
Ohita | 88.9 | 91.2 | 100 | |||||
Hamamatsu | 91.7 | 91.7 | 99.2 | 100 | ||||
Human parainfluenza Virus 1 | Discontiguous megablast for complete viral genomes (%) seq. ID AF457102.1 | |||||||
HPV1 (strain Washington/1964) | 75.2 | 73.9 | 74.5 | 74.6 | ||||
Porcine parainfluenza virus 1 | Discontiguous megablast for complete viral genomes (%) seq.ID NC_025402.1 | |||||||
PPV1 (strain S206N) | 71.15 | 75.1 | 70.5 | 71 |
Preparation
Sendai virus can be produced using specific pathogen-free (SPF) embryonated chicken eggs in accordance with the established protocol.[267] Caution should be exercised in adapting SeV for growth in cell culture for oncolytic research. One research study demonstrated that Sendai virus, adapted to grow in cell culture instead of chicken eggs, loses its oncolytic activity.[247][253]
Measurement of virus titer
The Sendai virus titer can be evaluated by serial end point 10x dilution assay of the virus-containing material in embryonated chicken eggs. This assay evaluates the final dilution that may cause a viral infection in infection in 50% of inoculated eggs. This EID50 assay is used to quantify titer for many viruses that can be grown in eggs.[268] The measurement of virus titer obtained from this assay is expressed as an embryonic infectious dose 50% (EID50). The SeV titer can also be assessed by using plaque assay in LLC-MK2 cells[269] and by serial end point 2x dilution hemagglutination assay (HA).[270] However, the HA test is less reliable than the EID50 or PFU tests because it does not always indicate the presence of a viable virus in a sample. The dead virus might demonstrate high HA titers.
Commercial availability
Sendai virus prep. for scientific research is available from Charles Rivers Laboratory. The produced virus is available in liquid,or lyophilised form of allontoic fluid,or sucrose gradient purified. Greek company Bioinnotech also produces the Sendai Virus for scientific research Sendai virus strain Z seed is available from ATCC,[271] Cantell strain is available from ATCC,[272] and from Charles Rivers Laboratory, Moscow strain is also available from ATCC.[273] Sendai Virus construct with Green Fluorescent Protein (SeV-GFP4) is available from ViraTree. Recombinant SeV proteins in E.Coli expression system for scientific studies including F (aa 26-500), M (aa 1-348), V (aa 1-384), L (aa 1-2228), W (aa 1-318), N (aa 1-524), C (aa 2-215) and M protein (aa 1-348) are available commercially in a form of recombinant DNA from Creative Biolabs Vaccine.The system for reprogramming of somatic cells into induced pluripotent stem cells is commercially available from ThermoFisher Scientific as CTS™ CytoTune™-iPS 2.1 Sendai Reprogramming Kit, Catalog number: A34546.
References
- Faísca P, Desmecht D (February 2007). "Sendai virus, the mouse parainfluenza type 1: a longstanding pathogen that remains up-to-date". Research in Veterinary Science. 82 (1): 115–25. doi:10.1016/j.rvsc.2006.03.009. PMID 16759680.
- Saga K, Kaneda Y (2015). "Oncolytic Sendai virus-based virotherapy for cancer: recent advances". Oncolytic Virotherapy. 4: 141–7. doi:10.2147/OV.S66419. PMC 4918391. PMID 27512677.
- Matveeva OV, Kochneva GV, Netesov SV, Onikienko SB, Chumakov PM (April 2015). "Mechanisms of Oncolysis by Paramyxovirus Sendai". Acta Naturae. 7 (2): 6–16. doi:10.32607/20758251-2015-7-2-6-16. PMC 4463408. PMID 26085940.
Material was copied from this source, which is available under a Creative Commons Attribution License. - Ilyinskaya GV, Mukhina EV, Soboleva AV, Matveeva OV, Chumakov PM (2018). "Oncolytic Sendai Virus Therapy of Canine Mast Cell Tumors (A Pilot Study)". Frontiers in Veterinary Science. 5: 116. doi:10.3389/fvets.2018.00116. PMC 5995045. PMID 29915788.
- Köhler G, Milstein C (August 1975). "Continuous cultures of fused cells secreting antibody of predefined specificity". Nature. 256 (5517): 495–7. Bibcode:1975Natur.256..495K. doi:10.1038/256495a0. PMID 1172191.
- Cassano, Amy; Rasmussen, Skye; Wolf, Felix R. (2012-01-01), Suckow, Mark A.; Stevens, Karla A.; Wilson, Ronald P. (eds.), "Chapter 31 - Viral Diseases", The Laboratory Rabbit, Guinea Pig, Hamster, and Other Rodents, American College of Laboratory Animal Medicine, Academic Press, pp. 821–837, ISBN 9780123809209, retrieved 2019-08-13
- MacLachlan, N. James; Dubovi, Edward J., eds. (2017-01-01), "Chapter 17 - Paramyxoviridae and Pneumoviridae", Fenner's Veterinary Virology (Fifth Edition), Academic Press, pp. 327–356, doi:10.1016/B978-0-12-800946-8.00017-9, ISBN 9780128009468, retrieved 2019-08-13
- Flecknell PA, Parry R, Needham JR, Ridley RM, Baker HF, Bowes P (April 1983). "Respiratory disease associated with parainfluenza Type I (Sendai) virus in a colony of marmosets (Callithrix jacchus)". Laboratory Animals. 17 (2): 111–3. doi:10.1258/002367783780959448. PMID 6306336.
- "The Laboratory Rabbit, Guinea Pig, Hamster, and Other Rodents | ScienceDirect". www.sciencedirect.com. Retrieved 2019-08-13.
- Nicklas W, Bleich A, Mähler M (2012-01-01). Hedrich HJ (ed.). Chapter 3.2 - Viral Infections of Laboratory Mice. The Laboratory Mouse (Second Edition). Academic Press. pp. 427–480. doi:10.1016/B978-0-12-382008-2.00019-2. ISBN 9780123820082.
- López CB, Yount JS, Hermesh T, Moran TM (May 2006). "Sendai virus infection induces efficient adaptive immunity independently of type I interferons". Journal of Virology. 80 (9): 4538–45. doi:10.1128/JVI.80.9.4538-4545.2006. PMC 1472017. PMID 16611914.
- Parker, J C; Whiteman, M D; Richter, C B (January 1978). "Susceptibility of inbred and outbred mouse strains to Sendai virus and prevalence of infection in laboratory rodents". Infection and Immunity. 19 (1): 123–130. ISSN 0019-9567. PMC 414057. PMID 203530.
- Brownstein, D. G.; Winkler, S. (April 1986). "Genetic resistance to lethal Sendai virus pneumonia: virus replication and interferon production in C57BL/6J and DBA/2J mice". Laboratory Animal Science. 36 (2): 126–129. ISSN 0023-6764. PMID 2422437.
- Simon, A. Y.; Moritoh, K.; Torigoe, D.; Asano, A.; Sasaki, N.; Agui, T. (December 2009). "Multigenic control of resistance to Sendai virus infection in mice". Infection, Genetics and Evolution. 9 (6): 1253–1259. doi:10.1016/j.meegid.2009.08.011. hdl:2115/42554. ISSN 1567-7257. PMID 19733691.
- Breider, M. A.; Adams, L. G.; Womack, J. E. (December 1987). "Influence of interferon in natural resistance of mice to Sendai virus pneumonia". American Journal of Veterinary Research. 48 (12): 1746–1750. ISSN 0002-9645. PMID 2449103.
- Sangster, M.; Smith, F. S.; Coleclough, C.; Hurwitz, J. L. (1995-09-10). "Human parainfluenza virus type 1 immunization of infant mice protects from subsequent Sendai virus infection". Virology. 212 (1): 13–19. doi:10.1006/viro.1995.1448. ISSN 0042-6822. PMID 7676623.
- Stone, Amy E. S.; Giguere, Steeve; Castleman, William L. (2003-11-07). "IL-12 reduces the severity of Sendai virus-induced bronchiolar inflammation and remodeling". Cytokine. 24 (3): 103–113. doi:10.1016/j.cyto.2003.07.005. ISSN 1043-4666. PMID 14581004.
- Koyama, A. Hajime; Irie, Hiroshi; Kato, Atsushi; Nagai, Yoshiyuki; Adachi, Akio (April 2003). "Virus multiplication and induction of apoptosis by Sendai virus: role of the C proteins". Microbes and Infection. 5 (5): 373–378. doi:10.1016/S1286-4579(03)00043-1. ISSN 1286-4579. PMID 12737992.
- Kiyotani, Katsuhiro; Sakaguchi, Takemasa; Kato, Atsushi; Nagai, Yoshiyuki; Yoshida, Tetsuya (2007-03-01). "Paramyxovirus Sendai virus V protein counteracts innate virus clearance through IRF-3 activation, but not via interferon, in mice". Virology. 359 (1): 82–91. doi:10.1016/j.virol.2006.08.053. ISSN 0042-6822. PMID 17027894.
- Irie, Takashi; Nagata, Natsuko; Igarashi, Tomoki; Okamoto, Isao; Sakaguchi, Takemasa (2010-05-19). "Conserved charged amino acids within Sendai virus C protein play multiple roles in the evasion of innate immune responses". PLOS ONE. 5 (5): e10719. Bibcode:2010PLoSO...510719I. doi:10.1371/journal.pone.0010719. ISSN 1932-6203. PMC 2873429. PMID 20502666.
- Kato, A.; Ohnishi, Y.; Kohase, M.; Saito, S.; Tashiro, M.; Nagai, Y. (April 2001). "Y2, the smallest of the Sendai virus C proteins, is fully capable of both counteracting the antiviral action of interferons and inhibiting viral RNA synthesis". Journal of Virology. 75 (8): 3802–3810. doi:10.1128/JVI.75.8.3802-3810.2001. ISSN 0022-538X. PMC 114871. PMID 11264369.
- Yamaguchi, Mayu; Kitagawa, Yoshinori; Zhou, Min; Itoh, Masae; Gotoh, Bin (2014-01-03). "An anti-interferon activity shared by paramyxovirus C proteins: inhibition of Toll-like receptor 7/9-dependent alpha interferon induction". FEBS Letters. 588 (1): 28–34. doi:10.1016/j.febslet.2013.11.015. ISSN 1873-3468. PMID 24269682.
- Andrejeva, J.; Childs, K. S.; Young, D. F.; Carlos, T. S.; Stock, N.; Goodbourn, S.; Randall, R. E. (2004-11-24). "The V proteins of paramyxoviruses bind the IFN-inducible RNA helicase, mda-5, and inhibit its activation of the IFN- promoter". Proceedings of the National Academy of Sciences. 101 (49): 17264–17269. doi:10.1073/pnas.0407639101. ISSN 0027-8424. PMC 535396. PMID 15563593.
- Childs, Kay; Stock, Nicola; Ross, Craig; Andrejeva, Jelena; Hilton, Louise; Skinner, Michael; Randall, Richard; Goodbourn, Stephen (March 2007). "mda-5, but not RIG-I, is a common target for paramyxovirus V proteins". Virology. 359 (1): 190–200. doi:10.1016/j.virol.2006.09.023. ISSN 0042-6822. PMID 17049367.
- Sánchez-Aparicio, Maria T.; Feinman, Leighland J.; García-Sastre, Adolfo; Shaw, Megan L. (2018-02-26). "Paramyxovirus V Proteins Interact with the RIG-I/TRIM25 Regulatory Complex and Inhibit RIG-I Signaling". Journal of Virology. 92 (6). doi:10.1128/JVI.01960-17. ISSN 0022-538X. PMC 5827389. PMID 29321315.
- Rochat, S.; Komada, H.; Kolakofsky, D. (July 1992). "Loss of V protein expression in human parainfluenza virus type 1 is not a recent event". Virus Research. 24 (2): 137–144. doi:10.1016/0168-1702(92)90002-q. ISSN 0168-1702. PMID 1326826.
- Oda, Kosuke; Matoba, Yasuyuki; Irie, Takashi; Kawabata, Ryoko; Fukushi, Masaya; Sugiyama, Masanori; Sakaguchi, Takemasa (November 2015). "Structural Basis of the Inhibition of STAT1 Activity by Sendai Virus C Protein". Journal of Virology. 89 (22): 11487–11499. doi:10.1128/JVI.01887-15. ISSN 1098-5514. PMC 4645678. PMID 26339056.
- Oda, Kosuke; Oda, Takashi; Matoba, Yasuyuki; Sato, Mamoru; Irie, Takashi; Sakaguchi, Takemasa (2017-10-04). "Structural analysis of the STAT1:STAT2 heterodimer revealed the mechanism of Sendai virus C protein–mediated blockade of type 1 interferon signaling". Journal of Biological Chemistry. 292 (48): 19752–19766. doi:10.1074/jbc.m117.786285. ISSN 0021-9258. PMC 5712616. PMID 28978648.
- Odkhuu, Erdenezaya; Komatsu, Takayuki; Naiki, Yoshikazu; Koide, Naoki; Yokochi, Takashi (November 2014). "Sendai virus C protein inhibits lipopolysaccharide-induced nitric oxide production through impairing interferon-β signaling". International Immunopharmacology. 23 (1): 267–272. doi:10.1016/j.intimp.2014.09.012. ISSN 1878-1705. PMID 25242386.
- Odkhuu, Erdenezaya; Komatsu, Takayuki; Koide, Naoki; Naiki, Yoshikazu; Takeuchi, Kenji; Tanaka, Yukie; Tsolmongyn, Bilegtsaikhan; Jambalganiin, Ulziisaikhan; Morita, Naoko (October 2018). "Sendai virus C protein limits NO production in infected RAW264.7 macrophages". Innate Immunity. 24 (7): 430–438. doi:10.1177/1753425918796619. ISSN 1753-4267. PMC 6830875. PMID 30189760.
- MacMicking, J.; Xie, Q. W.; Nathan, C. (1997). "Nitric oxide and macrophage function". Annual Review of Immunology. 15: 323–350. doi:10.1146/annurev.immunol.15.1.323. ISSN 0732-0582. PMID 9143691.
- Yokochi, Takashi; Gotoh, Bin; Morita, Naoko; Naiki, Yoshikazu; Koide, Naoki; Kitagawa, Yoshinori; Tanaka, Yukie; Komatsu, Takayuki (2018-10-01). "Sendai Virus V Protein Inhibits the Secretion of Interleukin-1β by Preventing NLRP3 Inflammasome Assembly". Journal of Virology. 92 (19): e00842–18. doi:10.1128/JVI.00842-18. ISSN 0022-538X. PMC 6146803. PMID 30021903.
- Kraft V, Meyer B (June 1986). "Diagnosis of murine infections in relation to test methods employed". Laboratory Animal Science. 36 (3): 271–6. PMID 3014210.
- Fox JG (2007). The Mouse in Biomedical Research, 2nd Edition. Burlington: Academic Press. pp. 281–309. doi:10.1016/B978-012369454-6/50039-X.
- Eaton GJ, Lerro A, Custer RP, Crane AR (August 1982). "Eradication of Sendai pneumonitis from a conventional mouse colony". Laboratory Animal Science. 32 (4): 384–6. PMID 6292576.
- Guenov, I.; Pavlov, N. (June 1972). "Study on parainfluenza virus type 1 isolated from pigs". Zentralblatt Fur Veterinarmedizin. Reihe B. Journal of Veterinary Medicine. Series B. 19 (6): 437–444. doi:10.1111/j.1439-0450.1972.tb00422.x. ISSN 0514-7166. PMID 4346239.
- Janke, B. H.; Paul, P. S.; Landgraf, J. G.; Halbur, P. G.; Huinker, C. D. (September 2001). "Paramyxovirus infection in pigs with interstitial pneumonia and encephalitis in the United States". Journal of Veterinary Diagnostic Investigation. 13 (5): 428–433. doi:10.1177/104063870101300513. ISSN 1040-6387. PMID 11580068.
- Lau, Susanna K. P.; Woo, Patrick C. Y.; Wu, Ying; Wong, Annette Y. P.; Wong, Beatrice H. L.; Lau, Candy C. Y.; Fan, Rachel Y. Y.; Cai, Jian-Piao; Tsoi, Hoi-Wah (October 2013). "Identification and characterization of a novel paramyxovirus, porcine parainfluenza virus 1, from deceased pigs". The Journal of General Virology. 94 (Pt 10): 2184–2190. doi:10.1099/vir.0.052985-0. ISSN 1465-2099. PMID 23918408.
- Palinski, Rachel M.; Chen, Zhenhai; Henningson, Jamie N.; Lang, Yuekun; Rowland, Raymond R. R.; Fang, Ying; Prickett, John; Gauger, Phillip C.; Hause, Ben M. (February 2016). "Widespread detection and characterization of porcine parainfluenza virus 1 in pigs in the USA". The Journal of General Virology. 97 (2): 281–286. doi:10.1099/jgv.0.000343. ISSN 1465-2099. PMID 26581410.
- Qiao, Dan; Janke, Bruce H.; Elankumaran, Subbiah (2009-04-01). "Molecular characterization of glycoprotein genes and phylogenetic analysis of two swine paramyxoviruses isolated from United States". Virus Genes. 39 (1): 53–65. doi:10.1007/s11262-009-0353-2. ISSN 0920-8569. PMID 19337823.
- Elankumaran, Subbiah; Janke, Bruce H.; Qiao, Dan (2010-01-15). "Complete Genome Sequence and Pathogenicity of Two Swine Parainfluenzavirus 3 Isolates from Pigs in the United States". Journal of Virology. 84 (2): 686–694. doi:10.1128/JVI.00847-09. ISSN 0022-538X. PMC 2798373. PMID 19906928.
- Slobod, Karen S.; Shenep, Jerry L.; Luján-Zilbermann, Jorge; Allison, Kim; Brown, Brita; Scroggs, Ruth Ann; Portner, Allen; Coleclough, Chris; Hurwitz, Julia L. (2004-08-13). "Safety and immunogenicity of intranasal murine parainfluenza virus type 1 (Sendai virus) in healthy human adults". Vaccine. 22 (23–24): 3182–3186. doi:10.1016/j.vaccine.2004.01.053. ISSN 0264-410X. PMID 15297072.
- Kuroya, M.; Ishida, N. (August 1953). "Newborn virus pneumonitis (type Sendai). II. The isolation of a new virus possessing hemagglutinin activity". Yokohama Medical Bulletin. 4 (4): 217–233. ISSN 0044-0531. PMID 13137076.
- Kuroya, M.; Ishida, N.; Shiratori, T. (June 1953). "Newborn virus pneumonitis (type Sendai). II. The isolation of a new virus". The Tohoku Journal of Experimental Medicine. 58 (1): 62. doi:10.1620/tjem.58.62. ISSN 0040-8727. PMID 13102529.
- Fukumi, H.; Nishikawa, F.; Kitayama, T. (August 1954). "A pneumotropic virus from mice causing hemagglutination". Japanese Journal of Medical Science & Biology. 7 (4): 345–363. doi:10.7883/yoken1952.7.345. ISSN 0021-5112. PMID 13232830.
- Ishida N, Homma M (1978). "Sendai virus". Advances in Virus Research. 23: 349–83. doi:10.1016/S0065-3527(08)60103-7. ISBN 9780120398232. PMID 219669.
- "Sendai virus | infectious agent". Encyclopedia Britannica. Retrieved 2019-08-26.
- Slobod KS, Shenep JL, Luján-Zilbermann J, Allison K, Brown B, Scroggs RA, et al. (August 2004). "Safety and immunogenicity of intranasal murine parainfluenza virus type 1 (Sendai virus) in healthy human adults". Vaccine. 22 (23–24): 3182–6. doi:10.1016/j.vaccine.2004.01.053. PMID 15297072.
- Skiadopoulos, Mario H.; Surman, Sonja R.; Riggs, Jeffrey M.; Elkins, William R.; St Claire, Marisa; Nishio, Machiko; Garcin, Dominique; Kolakofsky, Daniel; Collins, Peter L. (2002-05-25). "Sendai virus, a murine parainfluenza virus type 1, replicates to a level similar to human PIV1 in the upper and lower respiratory tract of African green monkeys and chimpanzees". Virology. 297 (1): 153–160. doi:10.1006/viro.2002.1416. ISSN 0042-6822. PMID 12083845.
- Bousse, Tatiana; Chambers, Raychel L.; Scroggs, Ruth Ann; Portner, Allen; Takimoto, Toru (October 2006). "Human parainfluenza virus type 1 but not Sendai virus replicates in human respiratory cells despite IFN treatment". Virus Research. 121 (1): 23–32. doi:10.1016/j.virusres.2006.03.012. ISSN 0168-1702. PMID 16677733.
- Hiscott, John; Lin, Rongtuan; Duguay, Delphine; tenOever, Benjamin R.; Grandvaux, Nathalie; Servant, Marc J. (2003-03-14). "Identification of the Minimal Phosphoacceptor Site Required for in Vivo Activation of Interferon Regulatory Factor 3 in Response to Virus and Double-stranded RNA". Journal of Biological Chemistry. 278 (11): 9441–9447. doi:10.1074/jbc.M209851200. ISSN 0021-9258. PMID 12524442.
- Peters, Kristi; Chattopadhyay, Saurabh; Sen, Ganes C. (April 2008). "IRF-3 Activation by Sendai Virus Infection Is Required for Cellular Apoptosis and Avoidance of Persistence". Journal of Virology. 82 (7): 3500–3508. doi:10.1128/JVI.02536-07. ISSN 0022-538X. PMC 2268502. PMID 18216110.
- Barnes, B. J.; Moore, P. A.; Pitha, P. M. (2001-06-29). "Virus-specific activation of a novel interferon regulatory factor, IRF-5, results in the induction of distinct interferon alpha genes". The Journal of Biological Chemistry. 276 (26): 23382–23390. doi:10.1074/jbc.M101216200. ISSN 0021-9258. PMID 11303025.
- Heylbroeck, Christophe; Balachandran, Siddharth; Servant, Marc J.; DeLuca, Carmela; Barber, Glen N.; Lin, Rongtuan; Hiscott, John (April 2000). "The IRF-3 Transcription Factor Mediates Sendai Virus-Induced Apoptosis". Journal of Virology. 74 (8): 3781–3792. doi:10.1128/jvi.74.8.3781-3792.2000. ISSN 0022-538X. PMC 111887. PMID 10729153.
- Mikkelsen, Susie S.; Jensen, Søren B.; Chiliveru, Srikanth; Melchjorsen, Jesper; Julkunen, Ilkka; Gaestel, Matthias; Arthur, J. Simon C.; Flavell, Richard A.; Ghosh, Sankar (2009-04-17). "RIG-I-mediated Activation of p38 MAPK Is Essential for Viral Induction of Interferon and Activation of Dendritic Cells". The Journal of Biological Chemistry. 284 (16): 10774–10782. doi:10.1074/jbc.M807272200. ISSN 0021-9258. PMC 2667765. PMID 19224920.
- Chen, Zhijian J.; Ea, Chee-Kwee; Sun, Lijun; Seth, Rashu B. (2005-09-09). "Identification and Characterization of MAVS, a Mitochondrial Antiviral Signaling Protein that Activates NF-κB and IRF3". Cell. 122 (5): 669–682. doi:10.1016/j.cell.2005.08.012. ISSN 0092-8674. PMID 16125763.
- Seth, Rashu B.; Sun, Lijun; Ea, Chee-Kwee; Chen, Zhijian J. (2005-09-09). "Identification and characterization of MAVS, a mitochondrial antiviral signaling protein that activates NF-kappaB and IRF 3". Cell. 122 (5): 669–682. doi:10.1016/j.cell.2005.08.012. ISSN 0092-8674. PMID 16125763.
- Gitlin, Leonid; Benoit, Loralyn; Song, Christina; Cella, Marina; Gilfillan, Susan; Holtzman, Michael J.; Colonna, Marco (2010-01-22). "Melanoma differentiation-associated gene 5 (MDA5) is involved in the innate immune response to Paramyxoviridae infection in vivo". PLoS Pathogens. 6 (1): e1000734. doi:10.1371/journal.ppat.1000734. ISSN 1553-7374. PMC 2809771. PMID 20107606.
- Milone, M. C.; Fitzgerald-Bocarsly, P. (1998-09-01). "The mannose receptor mediates induction of IFN-alpha in peripheral blood dendritic cells by enveloped RNA and DNA viruses". Journal of Immunology. 161 (5): 2391–2399. ISSN 0022-1767. PMID 9725235.
- Eloranta, M. L.; Sandberg, K.; Ricciardi-Castagnoli, P.; Lindahl, M.; Alm, G. V. (September 1997). "Production of interferon-alpha/beta by murine dendritic cell lines stimulated by virus and bacteria". Scandinavian Journal of Immunology. 46 (3): 235–241. doi:10.1046/j.1365-3083.1997.d01-120.x. ISSN 0300-9475. PMID 9315110.
- Iwasaki, Akiko; Mizushima, Noboru; Ramanathan, Balaji; Lund, Jennifer M.; Lee, Heung Kyu (2007-03-09). "Autophagy-Dependent Viral Recognition by Plasmacytoid Dendritic Cells". Science. 315 (5817): 1398–1401. Bibcode:2007Sci...315.1398L. doi:10.1126/science.1136880. ISSN 0036-8075. PMID 17272685.
- Izaguirre, Alexander; Barnes, Betsy J.; Amrute, Sheela; Yeow, Wen-Shuz; Megjugorac, Nicholas; Dai, Jihong; Feng, Di; Chung, Eugene; Pitha, Paula M. (December 2003). "Comparative analysis of IRF and IFN-alpha expression in human plasmacytoid and monocyte-derived dendritic cells". Journal of Leukocyte Biology. 74 (6): 1125–1138. doi:10.1189/jlb.0603255. ISSN 0741-5400. PMID 12960254.
- Shortman, Ken; Wu, Li; Hochrein, Hubertus; Pooley, Joanne; Vremec, David (2000-03-15). "CD4 and CD8 Expression by Dendritic Cell Subtypes in Mouse Thymus and Spleen". The Journal of Immunology. 164 (6): 2978–2986. doi:10.4049/jimmunol.164.6.2978. ISSN 0022-1767. PMID 10706685.
- Luber, Christian A.; Cox, Jürgen; Lauterbach, Henning; Fancke, Ben; Selbach, Matthias; Tschopp, Jurg; Akira, Shizuo; Wiegand, Marian; Hochrein, Hubertus (2010-02-26). "Quantitative proteomics reveals subset-specific viral recognition in dendritic cells". Immunity. 32 (2): 279–289. doi:10.1016/j.immuni.2010.01.013. ISSN 1097-4180. PMID 20171123.
- Kiener, Richard; Fleischmann, Markus; Wiegand, Marian Alexander; Lemmermann, Niels A. W.; Schwegler, Christiane; Kaufmann, Christine; Renzaho, Angelique; Thomas, Simone; Felder, Eva (2018-07-17). "Efficient Delivery of Human Cytomegalovirus T Cell Antigens by Attenuated Sendai Virus Vectors". Journal of Virology. 92 (15). doi:10.1128/JVI.00569-18. ISSN 0022-538X. PMC 6052310. PMID 29769344.
- Lappalainen, J; Rintahaka, J; Kovanen, P T; Matikainen, S; Eklund, K K (April 2013). "Intracellular RNA recognition pathway activates strong anti-viral response in human mast cells". Clinical and Experimental Immunology. 172 (1): 121–128. doi:10.1111/cei.12042. ISSN 0009-9104. PMC 3719938. PMID 23480192.
- Subramanian, Gayatri; Kuzmanovic, Teodora; Zhang, Ying; Peter, Cara Beate; Veleeparambil, Manoj; Chakravarti, Ritu; Sen, Ganes C.; Chattopadhyay, Saurabh (2018-01-30). "A new mechanism of interferon's antiviral action: Induction of autophagy, essential for paramyxovirus replication, is inhibited by the interferon stimulated gene, TDRD7". PLoS Pathogens. 14 (1): e1006877. doi:10.1371/journal.ppat.1006877. ISSN 1553-7366. PMC 5806901. PMID 29381763.
- Wetzel, Jaime L.; Fensterl, Volker; Sen, Ganes C. (December 2014). "Sendai virus pathogenesis in mice is prevented by Ifit2 and exacerbated by interferon". Journal of Virology. 88 (23): 13593–13601. doi:10.1128/JVI.02201-14. ISSN 1098-5514. PMC 4248979. PMID 25231314.
- Neerincx, Andreas; Lautz, Katja; Menning, Maureen; Kremmer, Elisabeth; Zigrino, Paola; Hösel, Marianna; Büning, Hildegard; Schwarzenbacher, Robert; Kufer, Thomas A. (2010-08-20). "A role for the human nucleotide-binding domain, leucine-rich repeat-containing family member NLRC5 in antiviral responses". The Journal of Biological Chemistry. 285 (34): 26223–26232. doi:10.1074/jbc.M110.109736. ISSN 1083-351X. PMC 2924034. PMID 20538593.
- Kanneganti, Thirumala-Devi; Body-Malapel, Mathilde; Amer, Amal; Park, Jong-Hwan; Whitfield, Joel; Franchi, Luigi; Taraporewala, Zenobia F.; Miller, David; Patton, John T. (2006-12-01). "Critical role for Cryopyrin/Nalp3 in activation of caspase-1 in response to viral infection and double-stranded RNA". The Journal of Biological Chemistry. 281 (48): 36560–36568. doi:10.1074/jbc.M607594200. ISSN 0021-9258. PMID 17008311.
- Park, Sangjun; Juliana, Christine; Hong, Sujeong; Datta, Pinaki; Hwang, Inhwa; Fernandes-Alnemri, Teresa; Yu, Je-Wook; Alnemri, Emad S. (2013-10-15). "The mitochondrial antiviral protein MAVS associates with NLRP3 and regulates its inflammasome activity". Journal of Immunology. 191 (8): 4358–4366. doi:10.4049/jimmunol.1301170. ISSN 1550-6606. PMC 3848201. PMID 24048902.
- Subramanian, Naeha; Natarajan, Kannan; Clatworthy, Menna R.; Wang, Ze; Germain, Ronald N. (April 2013). "The Adaptor MAVS Promotes NLRP3 Mitochondrial Localization and Inflammasome Activation". Cell. 153 (2): 348–361. doi:10.1016/j.cell.2013.02.054. ISSN 0092-8674. PMC 3632354. PMID 23582325.
- Ryan, Lisa Kathleen; Diamond, Gill (June 2017). "Modulation of Human β-Defensin-1 Production by Viruses". Viruses. 9 (6): 153. doi:10.3390/v9060153. ISSN 1999-4915. PMC 5490828. PMID 28635669.
- Ryan, Lisa K.; Dai, Jihong; Yin, Zhiwei; Megjugorac, Nicholas; Uhlhorn, Victoria; Yim, Sunghan; Schwartz, Kyell D.; Abrahams, Joshua M.; Diamond, Gill (August 2011). "Modulation of human beta-defensin-1 (hBD-1) in plasmacytoid dendritic cells (PDC), monocytes, and epithelial cells by influenza virus, Herpes simplex virus, and Sendai virus and its possible role in innate immunity". Journal of Leukocyte Biology. 90 (2): 343–356. doi:10.1189/jlb.0209079. ISSN 1938-3673. PMC 3133436. PMID 21551252.
- Nakanishi M, Otsu M (October 2012). "Development of Sendai virus vectors and their potential applications in gene therapy and regenerative medicine". Current Gene Therapy. 12 (5): 410–6. doi:10.2174/156652312802762518. PMC 3504922. PMID 22920683.
- Adderson E, Branum K, Sealy RE, Jones BG, Surman SL, Penkert R, et al. (March 2015). "Safety and immunogenicity of an intranasal Sendai virus-based human parainfluenza virus type 1 vaccine in 3- to 6-year-old children". Clinical and Vaccine Immunology. 22 (3): 298–303. doi:10.1128/CVI.00618-14. PMC 4340902. PMID 25552633.
- Kinoh H, Inoue M, Washizawa K, Yamamoto T, Fujikawa S, Tokusumi Y, et al. (July 2004). "Generation of a recombinant Sendai virus that is selectively activated and lyses human tumor cells expressing matrix metalloproteinases". Gene Therapy. 11 (14): 1137–45. doi:10.1038/sj.gt.3302272. PMID 15085175.
- Kinoh H, Inoue M (January 2008). "New cancer therapy using genetically-engineered oncolytic Sendai virus vector". Frontiers in Bioscience. 13 (13): 2327–34. doi:10.2741/2847. PMID 17981715.
- Tatsuta K, Tanaka S, Tajiri T, Shibata S, Komaru A, Ueda Y, et al. (February 2009). "Complete elimination of established neuroblastoma by synergistic action of gamma-irradiation and DCs treated with rSeV expressing interferon-beta gene". Gene Therapy. 16 (2): 240–51. doi:10.1038/gt.2008.161. PMID 18987675.
- Zimmermann M, Armeanu-Ebinger S, Bossow S, Lampe J, Smirnow I, Schenk A, et al. (2014). "Attenuated and protease-profile modified sendai virus vectors as a new tool for virotherapy of solid tumors". PLOS ONE. 9 (3): e90508. Bibcode:2014PLoSO...990508Z. doi:10.1371/journal.pone.0090508. PMC 3944018. PMID 24598703.
- Yonemitsu Y, Ueda Y, Kinoh H, Hasegawa M (January 2008). "Immunostimulatory virotherapy using recombinant Sendai virus as a new cancer therapeutic regimen". Frontiers in Bioscience. 13 (13): 1892–8. doi:10.2741/2809. PMID 17981677.
- Iwadate Y, Inoue M, Saegusa T, Tokusumi Y, Kinoh H, Hasegawa M, et al. (May 2005). "Recombinant Sendai virus vector induces complete remission of established brain tumors through efficient interleukin-2 gene transfer in vaccinated rats". Clinical Cancer Research. 11 (10): 3821–7. doi:10.1158/1078-0432.CCR-04-1485. PMID 15897582.
- Tanaka M, Shimbo T, Kikuchi Y, Matsuda M, Kaneda Y (April 2010). "Sterile alpha motif containing domain 9 is involved in death signaling of malignant glioma treated with inactivated Sendai virus particle (HVJ-E) or type I interferon". International Journal of Cancer. 126 (8): 1982–1991. doi:10.1002/ijc.24965. PMID 19830690.
- Qian M, Tan HM, Yu N, Wang T, Zhang Q (April 2018). "Inactivated Sendai Virus Induces ROS-dependent Apoptosis and Autophagy in Human Prostate Cancer Cells". Biomedical and Environmental Sciences. 31 (4): 280–289. doi:10.3967/bes2018.036. PMID 29773091.
- Kawaguchi Y, Miyamoto Y, Inoue T, Kaneda Y (May 2009). "Efficient eradication of hormone-resistant human prostate cancers by inactivated Sendai virus particle". International Journal of Cancer. 124 (10): 2478–87. doi:10.1002/ijc.24234. PMID 19173282.
- Kurooka M, Kaneda Y (January 2007). "Inactivated Sendai virus particles eradicate tumors by inducing immune responses through blocking regulatory T cells". Cancer Research. 67 (1): 227–36. doi:10.1158/0008-5472.CAN-06-1615. PMID 17210703.
- Fujihara A, Kurooka M, Miki T, Kaneda Y (January 2008). "Intratumoral injection of inactivated Sendai virus particles elicits strong antitumor activity by enhancing local CXCL10 expression and systemic NK cell activation". Cancer Immunology, Immunotherapy. 57 (1): 73–84. doi:10.1007/s00262-007-0351-y. PMID 17602226.
- Nishikawa T, Tung LY, Kaneda Y (December 2014). "Systemic administration of platelets incorporating inactivated Sendai virus eradicates melanoma in mice". Molecular Therapy. 22 (12): 2046–55. doi:10.1038/mt.2014.128. PMC 4429689. PMID 25023327.
- Zhang Q, Yuan WF, Zhai GQ, Zhu SY, Xue ZF, Zhu HF, Xu XM (October 2012). "Inactivated Sendai virus suppresses murine melanoma growth by inducing host immune responses and down-regulating β-catenin expression". Biomedical and Environmental Sciences. 25 (5): 509–16. doi:10.3967/0895-3988.2012.05.003. PMID 23122307.
- Saga K, Tamai K, Yamazaki T, Kaneda Y (February 2013). "Systemic administration of a novel immune-stimulatory pseudovirion suppresses lung metastatic melanoma by regionally enhancing IFN-γ production". Clinical Cancer Research. 19 (3): 668–79. doi:10.1158/1078-0432.CCR-12-1947. PMID 23251005.
- Wheelock, E. F.; Dingle, J. H. (1964-09-24). "Observations on the Repeated Administration of Viruses to a Patient with Acute Leukemia". The New England Journal of Medicine. 271 (13): 645–651. doi:10.1056/NEJM196409242711302. ISSN 0028-4793. PMID 14170843.
- Zainutdinov, Sergei S; Kochneva, Galina V; Netesov, Sergei V; Chumakov, Peter M.; Matveeva, Olga V (July 2019). "
Directed evolution as a tool for the selection of oncolytic RNA viruses with desired phenotypes
". Oncolytic Virotherapy. 8: 9–26. doi:10.2147/ov.s176523. ISSN 2253-1572. PMC 6636189. PMID 31372363. - Zainutdinov, Sergei S.; Tikunov, Artem Y.; Matveeva, Olga V.; Netesov, Sergei V.; Kochneva, Galina V. (2016-08-11). "Complete Genome Sequence of the Oncolytic Sendai virus Strain Moscow". Genome Announcements. 4 (4). doi:10.1128/genomeA.00818-16. ISSN 2169-8287. PMC 4982289. PMID 27516510.
- Treatment of advanced metastatic cancers with oncolytic Sendai virus, retrieved 2019-08-21
- , "Method for cancer immunotherapy and pharmaceutical compositions based on oncolytic non-pathogenic Sendai virus", issued 2013-11-21
- Gao, Wei; Liang, Jin-xiao; Liang, Yong (May 2016). "Clinicopathological and prognostic significance of sialyl Lewis X overexpression in patients with cancer: a meta-analysis". OncoTargets and Therapy. 9: 3113–25. doi:10.2147/ott.s102389. ISSN 1178-6930. PMC 4888715. PMID 27307752.
- Noguchi, Masaaki; Sato, Naotake; Sugimori, Hiroki; Mori, Kiyoshi; Oshimi, Kazuo (October 2001). "A minor E-selectin ligand, CD65, is critical for extravascular infiltration of acute myeloid leukemia cells". Leukemia Research. 25 (10): 847–853. doi:10.1016/s0145-2126(01)00036-4. ISSN 0145-2126. PMID 11532516.
- "KEGG GLYCAN: G00111". www.genome.jp. Retrieved 2019-08-13.
- Liang, Y.-J.; Ding, Y.; Levery, S. B.; Lobaton, M.; Handa, K.; Hakomori, S.-i. (2013-03-11). "Differential expression profiles of glycosphingolipids in human breast cancer stem cells vs. cancer non-stem cells". Proceedings of the National Academy of Sciences. 110 (13): 4968–4973. Bibcode:2013PNAS..110.4968L. doi:10.1073/pnas.1302825110. ISSN 0027-8424. PMC 3612608. PMID 23479608.
- Chambers, Michael. "ChemIDplus - 71833-57-3 - OWMXULOUTAAVIX-HNZIOFRCSA-N - Sialosylparagloboside - Similar structures search, synonyms, formulas, resource links, and other chemical information". chem.nlm.nih.gov. Retrieved 2019-08-13.
- Okegawa, Takatsugu (2018), "Detection of Circulating Tumor Cells in Castration-Resistant Prostate Cancer", Hormone Therapy and Castration Resistance of Prostate Cancer, Springer Singapore, pp. 299–305, doi:10.1007/978-981-10-7013-6_30, ISBN 9789811070129
- Hatano, Koji; Miyamoto, Yasuhide; Nonomura, Norio; Kaneda, Yasufumi (2011-04-13). "Expression of gangliosides, GD1a, and sialyl paragloboside is regulated by NF-κB-dependent transcriptional control of α2,3-sialyltransferase I, II, and VI in human castration-resistant prostate cancer cells". International Journal of Cancer. 129 (8): 1838–1847. doi:10.1002/ijc.25860. ISSN 0020-7136. PMID 21165949.
- Hamasaki, Hiroko; Aoyagi, Masaru; Kasama, Takeshi; Handa, Shizuo; Hirakawa, Kimiyoshi; Taki, Takao (January 1999). "GT1b in human metastatic brain tumors: GT1b as a brain metastasis-associated ganglioside". Biochimica et Biophysica Acta (BBA) - Molecular and Cell Biology of Lipids. 1437 (1): 93–99. doi:10.1016/s1388-1981(98)00003-1. ISSN 1388-1981. PMID 9931455.
- Hidalgo, I. J.; Raub, T. J.; Borchardt, R. T. (March 1989). "Characterization of the human colon carcinoma cell line (Caco-2) as a model system for intestinal epithelial permeability". Gastroenterology. 96 (3): 736–749. doi:10.1016/0016-5085(89)90897-4. ISSN 0016-5085. PMID 2914637.
- "Cell atlas - TMPRSS2 - The Human Protein Atlas". www.proteinatlas.org. Retrieved 2019-08-20.
- "Expression of TPSB2 in cancer - Summary - The Human Protein Atlas". www.proteinatlas.org. Retrieved 2019-08-20.
- "Cell atlas - TPSB2 - The Human Protein Atlas". www.proteinatlas.org. Retrieved 2019-08-20.
- Nilsson, G.; Blom, T.; Kusche-Gullberg, M.; Kjellén, L.; Butterfield, J. H.; Sundström, C.; Nilsson, K.; Hellman, L. (May 1994). "Phenotypic characterization of the human mast-cell line HMC-1". Scandinavian Journal of Immunology. 39 (5): 489–498. doi:10.1111/j.1365-3083.1994.tb03404.x. ISSN 0300-9475. PMID 8191224.
- Martin, P.; Papayannopoulou, T. (1982-06-11). "HEL cells: a new human erythroleukemia cell line with spontaneous and induced globin expression". Science. 216 (4551): 1233–1235. Bibcode:1982Sci...216.1233M. doi:10.1126/science.6177045. ISSN 0036-8075. PMID 6177045.
- "Expression of PLG in cancer - Summary - The Human Protein Atlas". www.proteinatlas.org. Retrieved 2019-08-20.
- "Expression of F10 in cancer - Summary - The Human Protein Atlas". www.proteinatlas.org. Retrieved 2019-08-30.
- Hasegawa, M.; Nagai, Y.; Iida, A.; Tokusumi, Y.; Fujikawa, S.; Yamamoto, T.; Washizawa, K.; Inoue, M.; Kinoh, H. (July 2004). "Generation of a recombinant Sendai virus that is selectively activated and lyses human tumor cells expressing matrix metalloproteinases". Gene Therapy. 11 (14): 1137–1145. doi:10.1038/sj.gt.3302272. ISSN 1476-5462. PMID 15085175.
- Matveeva, Olga V.; Chumakov, Peter M. (November 2018). "Defects in interferon pathways as potential biomarkers of sensitivity to oncolytic viruses". Reviews in Medical Virology. 28 (6): e2008. doi:10.1002/rmv.2008. ISSN 1099-1654. PMID 30209859.
- Bedsaul, Jacquelyn R.; Zaritsky, Luna A.; Zoon, Kathryn C. (2016-11-01). "Type I Interferon-Mediated Induction of Antiviral Genes and Proteins Fails to Protect Cells from the Cytopathic Effects of Sendai Virus Infection". Journal of Interferon & Cytokine Research. 36 (11): 652–665. doi:10.1089/jir.2016.0051. ISSN 1079-9907. PMC 5105340. PMID 27508859.
- Mandhana, Roli; Horvath, Curt M. (2018-11-14). "Sendai Virus Infection Induces Expression of Novel RNAs in Human Cells". Scientific Reports. 8 (1): 16815. Bibcode:2018NatSR...816815M. doi:10.1038/s41598-018-35231-8. ISSN 2045-2322. PMC 6235974. PMID 30429577.
- Ebert, Oliver; Shinozaki, Katsunori; Kournioti, Chryssanthi; Park, Man-Seong; García-Sastre, Adolfo; Woo, Savio L. C. (2004-05-01). "Syncytia induction enhances the oncolytic potential of vesicular stomatitis virus in virotherapy for cancer". Cancer Research. 64 (9): 3265–3270. doi:10.1158/0008-5472.CAN-03-3753. ISSN 0008-5472. PMID 15126368.
- Nakamori, Mikihito; Fu, Xinping; Meng, Feng; Jin, Aiwu; Tao, Lihua; Bast, Robert C.; Zhang, Xiaoliu (July 2003). "Effective therapy of metastatic ovarian cancer with an oncolytic herpes simplex virus incorporating two membrane fusion mechanisms". Clinical Cancer Research. 9 (7): 2727–2733. ISSN 1078-0432. PMID 12855653.
- Altomonte, Jennifer; Marozin, Sabrina; Schmid, Roland M.; Ebert, Oliver (February 2010). "Engineered newcastle disease virus as an improved oncolytic agent against hepatocellular carcinoma". Molecular Therapy. 18 (2): 275–284. doi:10.1038/mt.2009.231. ISSN 1525-0024. PMC 2839313. PMID 19809404.
- Tai, Jiayu A.; Chang, Chin Yang; Nishikawa, Tomoyuki; Kaneda, Yasufumi (2019-08-06). "Cancer immunotherapy using the Fusion gene of Sendai virus". Cancer Gene Therapy. doi:10.1038/s41417-019-0126-6. ISSN 1476-5500. PMID 31383952.
- Bateman, A.; Bullough, F.; Murphy, S.; Emiliusen, L.; Lavillette, D.; Cosset, F. L.; Cattaneo, R.; Russell, S. J.; Vile, R. G. (2000-03-15). "Fusogenic membrane glycoproteins as a novel class of genes for the local and immune-mediated control of tumor growth". Cancer Research. 60 (6): 1492–1497. ISSN 0008-5472. PMID 10749110.
- Galanis, E.; Bateman, A.; Johnson, K.; Diaz, R. M.; James, C. D.; Vile, R.; Russell, S. J. (2001-05-01). "Use of viral fusogenic membrane glycoproteins as novel therapeutic transgenes in gliomas". Human Gene Therapy. 12 (7): 811–821. doi:10.1089/104303401750148766. ISSN 1043-0342. PMID 11339897.
- Lin, E.-H.; Salon, C.; Brambilla, E.; Lavillette, D.; Szecsi, J.; Cosset, F.-L.; Coll, J.-L. (April 2010). "Fusogenic membrane glycoproteins induce syncytia formation and death in vitro and in vivo: a potential therapy agent for lung cancer". Cancer Gene Therapy. 17 (4): 256–265. doi:10.1038/cgt.2009.74. ISSN 1476-5500. PMID 19893593.
- Hua, J.; Liao, M. J.; Rashidbaigi, A. (July 1996). "Cytokines induced by Sendai virus in human peripheral blood leukocytes". Journal of Leukocyte Biology. 60 (1): 125–128. doi:10.1002/jlb.60.1.125. ISSN 0741-5400. PMID 8699116.
- Costas, M. A.; Mella, D.; Criscuolo, M.; Díaz, A.; Finkielman, S.; Nahmod, V. E.; Arzt, E. (December 1993). "Superinduction of mitogen-stimulated interferon-gamma production and other lymphokines by Sendai virus". Journal of Interferon Research. 13 (6): 407–412. doi:10.1089/jir.1993.13.407. ISSN 0197-8357. PMID 8151134.
- Zidovec, S.; Mazuran, R. (February 1999). "Sendai virus induces various cytokines in human peripheral blood leukocytes: different susceptibility of cytokine molecules to low pH". Cytokine. 11 (2): 140–143. doi:10.1006/cyto.1998.0411. ISSN 1043-4666. PMID 10089135.
- Lin, H. Y.; Davis, P. J.; Thacore, H. R. (December 1991). "Production of human interferon-beta by Sendai virus and poly(rI).poly(rC): inhibition by neomycin". Journal of Interferon Research. 11 (6): 365–369. doi:10.1089/jir.1991.11.365. ISSN 0197-8357. PMID 1666117.
- Cantell, K.; Hirvonen, S.; Kauppinen, H. L.; Myllylä, G. (1981). "Production of interferon in human leukocytes from normal donors with the use of Sendai virus". Methods in Enzymology. 78 (Pt A): 29–38. doi:10.1016/0076-6879(81)78094-7. ISSN 0076-6879. PMID 6173603.
- Nyman, T A; Tölö, H; Parkkinen, J; Kalkkinen, N (1998-01-15). "Identification of nine interferon-alpha subtypes produced by Sendai virus-induced human peripheral blood leucocytes". Biochemical Journal. 329 (Pt 2): 295–302. doi:10.1042/bj3290295. ISSN 0264-6021. PMC 1219044. PMID 9425112.
- Suzuki, Hayato; Kurooka, Masayuki; Hiroaki, Yoko; Fujiyoshi, Yoshinori; Kaneda, Yasufumi (2008-04-16). "Sendai virus F glycoprotein induces IL-6 production in dendritic cells in a fusion-independent manner". FEBS Letters. 582 (9): 1325–1329. doi:10.1016/j.febslet.2008.03.011. ISSN 0014-5793. PMID 18358837.
- Zeng J, Fournier P, Schirrmacher V (May 2002). "Induction of interferon-alpha and tumor necrosis factor-related apoptosis-inducing ligand in human blood mononuclear cells by hemagglutinin-neuraminidase but not F protein of Newcastle disease virus". Virology. 297 (1): 19–30. doi:10.1006/viro.2002.1413. PMID 12083832.
- Ito Y, Hosaka Y (March 1983). "Component(s) of Sendai virus that can induce interferon in mouse spleen cells". Infection and Immunity. 39 (3): 1019–23. PMC 348058. PMID 6301988.
- Donnelly, Raymond P.; Kotenko, Sergei V. (August 2010). "Interferon-lambda: a new addition to an old family". Journal of Interferon & Cytokine Research. 30 (8): 555–564. doi:10.1089/jir.2010.0078. ISSN 1557-7465. PMC 2925029. PMID 20712453.
- Yin, Zhiwei; Dai, Jihong; Deng, Jing; Sheikh, Faruk; Natalia, Mahwish; Shih, Tiffany; Lewis-Antes, Anita; Amrute, Sheela B.; Garrigues, Ursula (2012-09-15). "Type III IFNs are produced by and stimulate human plasmacytoid dendritic cells". Journal of Immunology. 189 (6): 2735–2745. doi:10.4049/jimmunol.1102038. ISSN 0022-1767. PMC 3579503. PMID 22891284.
- Costas, M. A.; Mella, D.; Criscuolo, M.; Díaz, A.; Finkielman, S.; Nahmod, V. E.; Arzt, E. (December 1993). "Superinduction of mitogen-stimulated interferon-gamma production and other lymphokines by Sendai virus". Journal of Interferon Research. 13 (6): 407–412. doi:10.1089/jir.1993.13.407. ISSN 0197-8357. PMID 8151134.
- Hua, J.; Liao, M. J.; Rashidbaigi, A. (July 1996). "Cytokines induced by Sendai virus in human peripheral blood leukocytes". Journal of Leukocyte Biology. 60 (1): 125–128. doi:10.1002/jlb.60.1.125. ISSN 0741-5400. PMID 8699116.
- Suzuki, Hayato; Kurooka, Masayuki; Hiroaki, Yoko; Fujiyoshi, Yoshinori; Kaneda, Yasufumi (2008-03-20). "Sendai virus F glycoprotein induces IL-6 production in dendritic cells in a fusion-independent manner". FEBS Letters. 582 (9): 1325–1329. doi:10.1016/j.febslet.2008.03.011. ISSN 0014-5793. PMID 18358837.
- Ittah, Marc; Miceli-Richard, Corinne; Lebon, Pierre; Pallier, Coralie; Lepajolec, Christine; Mariette, Xavier (2011). "Induction of B cell-activating factor by viral infection is a general phenomenon, but the types of viruses and mechanisms depend on cell type". Journal of Innate Immunity. 3 (2): 200–207. doi:10.1159/000321194. ISSN 1662-8128. PMID 21051868.
- Johansson, E.; Domeika, K.; Berg, M.; Alm, G. V.; Fossum, C. (2003-02-10). "Characterisation of porcine monocyte-derived dendritic cells according to their cytokine profile". Veterinary Immunology and Immunopathology. 91 (3–4): 183–197. doi:10.1016/s0165-2427(02)00310-0. ISSN 0165-2427. PMID 12586481.
- Li, Simin; Nishikawa, Tomoyuki; Kaneda, Yasufumi (December 2017). "Inactivated Sendai virus particle upregulates cancer cell expression of intercellular adhesion molecule-1 and enhances natural killer cell sensitivity on cancer cells". Cancer Science. 108 (12): 2333–2341. doi:10.1111/cas.13408. ISSN 1349-7006. PMC 5715349. PMID 28945328.
- Pearlstein, E.; Salk, P. L.; Yogeeswaran, G.; Karpatkin, S. (July 1980). "Correlation between spontaneous metastatic potential, platelet-aggregating activity of cell surface extracts, and cell surface sialylation in 10 metastatic-variant derivatives of a rat renal sarcoma cell line". Proceedings of the National Academy of Sciences of the United States of America. 77 (7): 4336–4339. Bibcode:1980PNAS...77.4336P. doi:10.1073/pnas.77.7.4336. ISSN 0027-8424. PMC 349829. PMID 6933486.
- Yogeeswaran, G.; Salk, P. L. (1981-06-26). "Metastatic potential is positively correlated with cell surface sialylation of cultured murine tumor cell lines". Science. 212 (4502): 1514–1516. Bibcode:1981Sci...212.1514Y. doi:10.1126/science.7233237. ISSN 0036-8075. PMID 7233237.
- Benedetto, A.; Elia, G.; Sala, A.; Belardelli, F. (1989-01-15). "Hyposialylation of high-molecular-weight membrane glycoproteins parallels the loss of metastatic potential in wheat-germ agglutinin-resistant Friend leukemia cells". International Journal of Cancer. 43 (1): 126–133. doi:10.1002/ijc.2910430124. ISSN 0020-7136. PMID 2910824.
- Collard, J. G.; Schijven, J. F.; Bikker, A.; La Riviere, G.; Bolscher, J. G.; Roos, E. (July 1986). "Cell surface sialic acid and the invasive and metastatic potential of T-cell hybridomas". Cancer Research. 46 (7): 3521–3527. ISSN 0008-5472. PMID 3486712.
- Passaniti, A.; Hart, G. W. (1988-06-05). "Cell surface sialylation and tumor metastasis. Metastatic potential of B16 melanoma variants correlates with their relative numbers of specific penultimate oligosaccharide structures". The Journal of Biological Chemistry. 263 (16): 7591–7603. ISSN 0021-9258. PMID 3372501.
- Bresalier, R. S.; Rockwell, R. W.; Dahiya, R.; Duh, Q. Y.; Kim, Y. S. (1990-02-15). "Cell surface sialoprotein alterations in metastatic murine colon cancer cell lines selected in an animal model for colon cancer metastasis". Cancer Research. 50 (4): 1299–1307. ISSN 0008-5472. PMID 2297775.
- Hsu, Chi-Cheng; Lin, Tzu-Wen; Chang, Wei-Wei; Wu, Chi-Yue; Lo, Wan-Hsia; Wang, Peng-Hui; Tsai, Ying-Chieh (February 2005). "Soyasaponin-I-modified invasive behavior of cancer by changing cell surface sialic acids". Gynecologic Oncology. 96 (2): 415–422. doi:10.1016/j.ygyno.2004.10.010. ISSN 0090-8258. PMID 15661230.
- Chang, Wei-Wei; Yu, Chia-Yu; Lin, Tzu-Wen; Wang, Peng-Hui; Tsai, Ying-Chieh (2006-03-10). "Soyasaponin I decreases the expression of alpha2,3-linked sialic acid on the cell surface and suppresses the metastatic potential of B16F10 melanoma cells". Biochemical and Biophysical Research Communications. 341 (2): 614–619. doi:10.1016/j.bbrc.2005.12.216. ISSN 0006-291X. PMID 16427612.
- Chiang, Chi-Hsiang; Wang, Chie-Hong; Chang, Hui-Chiu; More, Shivaji V.; Li, Wen-Shan; Hung, Wen-Chun (May 2010). "A novel sialyltransferase inhibitor AL10 suppresses invasion and metastasis of lung cancer cells by inhibiting integrin-mediated signaling". Journal of Cellular Physiology. 223 (2): 492–499. doi:10.1002/jcp.22068. ISSN 1097-4652. PMID 20112294.
- Cohen, Merav; Elkabets, Moshe; Perlmutter, Michal; Porgador, Angel; Voronov, Elena; Apte, Ron N.; Lichtenstein, Rachel G. (2010-11-15). "Sialylation of 3-methylcholanthrene-induced fibrosarcoma determines antitumor immune responses during immunoediting". Journal of Immunology. 185 (10): 5869–5878. doi:10.4049/jimmunol.1001635. ISSN 1550-6606. PMID 20956342.
- Bossart, Katharine N.; Fusco, Deborah L.; Broder, Christopher C. (2013). "Paramyxovirus entry". Advances in Experimental Medicine and Biology. 790: 95–127. doi:10.1007/978-1-4614-7651-1_6. ISBN 978-1-4614-7650-4. ISSN 0065-2598. PMID 23884588.
- Powell, L. D.; Whiteheart, S. W.; Hart, G. W. (1987-07-01). "Cell surface sialic acid influences tumor cell recognition in the mixed lymphocyte reaction". Journal of Immunology. 139 (1): 262–270. ISSN 0022-1767. PMID 2953814.
- Tyagarajan, K.; Forte, J. G.; Townsend, R. R. (January 1996). "Exoglycosidase purity and linkage specificity: assessment using oligosaccharide substrates and high-pH anion-exchange chromatography with pulsed amperometric detection". Glycobiology. 6 (1): 83–93. doi:10.1093/glycob/6.1.83. ISSN 0959-6658. PMID 8991514.
- Drzeniek, R.; Gauhe, A. (1970-02-20). "Differences in substrate specificity of myxovirus neuraminidases". Biochemical and Biophysical Research Communications. 38 (4): 651–656. doi:10.1016/0006-291x(70)90630-3. ISSN 0006-291X. PMID 5443707.
- Brostrom, M. A.; Bruening, G.; Bankowski, R. A. (December 1971). "Comparison of neuraminidases of paramyxoviruses with immunologically dissimilar hemagglutinins". Virology. 46 (3): 856–865. doi:10.1016/0042-6822(71)90086-9. ISSN 0042-6822. PMID 4332979.
- Fujihara, Atsuko; Kurooka, Masayuki; Miki, Tsuneharu; Kaneda, Yasufumi (January 2008). "Intratumoral injection of inactivated Sendai virus particles elicits strong antitumor activity by enhancing local CXCL10 expression and systemic NK cell activation". Cancer Immunology, Immunotherapy. 57 (1): 73–84. doi:10.1007/s00262-007-0351-y. ISSN 0340-7004. PMID 17602226.
- Jarahian, Mostafa; Watzl, Carsten; Fournier, Philippe; Arnold, Annette; Djandji, Dominik; Zahedi, Sarah; Cerwenka, Adelheid; Paschen, Annette; Schirrmacher, Volker (August 2009). "Activation of natural killer cells by newcastle disease virus hemagglutinin-neuraminidase". Journal of Virology. 83 (16): 8108–8121. doi:10.1128/JVI.00211-09. ISSN 1098-5514. PMC 2715740. PMID 19515783.
- Arnon, T. I.; Lev, M.; Katz, G.; Chernobrov, Y.; Porgador, A.; Mandelboim, O. (September 2001). "Recognition of viral hemagglutinins by NKp44 but not by NKp30". European Journal of Immunology. 31 (9): 2680–2689. doi:10.1002/1521-4141(200109)31:9<2680::AID-IMMU2680>3.0.CO;2-A. ISSN 0014-2980. PMID 11536166.
- Schirrmacher, V.; Haas, C.; Bonifer, R.; Ertel, C. (July 1997). "Virus potentiation of tumor vaccine T-cell stimulatory capacity requires cell surface binding but not infection". Clinical Cancer Research. 3 (7): 1135–1148. ISSN 1078-0432. PMID 9815793.
- Harada, Yui; Yonemitsu, Yoshikazu (2011-06-01). "Dramatic improvement of DC-based immunotherapy against various malignancies". Frontiers in Bioscience (Landmark Edition). 16: 2233–2242. doi:10.2741/3850. ISSN 1093-4715. PMID 21622173.
- Shibata, Satoko; Okano, Shinji; Yonemitsu, Yoshikazu; Onimaru, Mitsuho; Sata, Shihoko; Nagata-Takeshita, Hiroko; Inoue, Makoto; Zhu, Tsugumine; Hasegawa, Mamoru (2006-09-15). "Induction of efficient antitumor immunity using dendritic cells activated by recombinant Sendai virus and its modulation by exogenous IFN-beta gene". Journal of Immunology. 177 (6): 3564–3576. doi:10.4049/jimmunol.177.6.3564. ISSN 0022-1767. PMID 16951315.
- Okano, Shinji; Yonemitsu, Yoshikazu; Shirabe, Ken; Kakeji, Yoshihiro; Maehara, Yoshihiko; Harada, Mamoru; Yoshikai, Yasunobu; Inoue, Makoto; Hasegawa, Mamoru (2011-02-01). "Provision of continuous maturation signaling to dendritic cells by RIG-I-stimulating cytosolic RNA synthesis of Sendai virus". Journal of Immunology. 186 (3): 1828–1839. doi:10.4049/jimmunol.0901641. ISSN 1550-6606. PMID 21187441.
- Sugiyama, Masahiko; Kakeji, Yoshihiro; Tsujitani, Shunichi; Harada, Yui; Onimaru, Mitsuho; Yoshida, Kumi; Tanaka, Sakura; Emi, Yasunori; Morita, Masaru (March 2011). "Antagonism of VEGF by genetically engineered dendritic cells is essential to induce antitumor immunity against malignant ascites". Molecular Cancer Therapeutics. 10 (3): 540–549. doi:10.1158/1535-7163.MCT-10-0479. ISSN 1538-8514. PMID 21209070.
- Yoneyama, Yasuo; Ueda, Yasuji; Akutsu, Yasunori; Matsunaga, Akinao; Shimada, Hideaki; Kato, Tomonori; Kubota-Akizawa, Megumi; Okano, Shinji; Shibata, Satoko (2007-03-30). "Development of immunostimulatory virotherapy using non-transmissible Sendai virus-activated dendritic cells". Biochemical and Biophysical Research Communications. 355 (1): 129–135. doi:10.1016/j.bbrc.2007.01.132. ISSN 0006-291X. PMID 17292867.
- Yonemitsu, Yoshikazu; Ueda, Yasuji; Kinoh, Hiroaki; Hasegawa, Mamoru (2008-01-01). "Immunostimulatory virotherapy using recombinant Sendai virus as a new cancer therapeutic regimen". Frontiers in Bioscience. 13 (13): 1892–1898. doi:10.2741/2809. ISSN 1093-9946. PMID 17981677.
- Komaru, Atsushi; Ueda, Yasuji; Furuya, Aki; Tanaka, Sakura; Yoshida, Kumi; Kato, Tomonori; Kinoh, Hiroaki; Harada, Yui; Suzuki, Hiroyoshi (2009-10-01). "Sustained and NK/CD4+ T cell-dependent efficient prevention of lung metastasis induced by dendritic cells harboring recombinant Sendai virus". Journal of Immunology. 183 (7): 4211–4219. doi:10.4049/jimmunol.0803845. ISSN 1550-6606. PMID 19734206.
- Kato, Tomonori; Ueda, Yasuji; Kinoh, Hiroaki; Yoneyama, Yasuo; Matsunaga, Akinao; Komaru, Atsushi; Harada, Yui; Suzuki, Hiroyoshi; Komiya, Akira (November 2010). "RIG-I helicase-independent pathway in sendai virus-activated dendritic cells is critical for preventing lung metastasis of AT6.3 prostate cancer". Neoplasia (New York, N.Y.). 12 (11): 906–914. doi:10.1593/neo.10732. ISSN 1476-5586. PMC 2978913. PMID 21076616.
- Hosoya, Noriaki; Miura, Toshiyuki; Kawana‐Tachikawa, Ai; Koibuchi, Tomohiko; Shioda, Tatsuo; Odawara, Takashi; Nakamura, Tetsuya; Kitamura, Yoshihiro; Kano, Munehide (2008). "Comparison between Sendai virus and adenovirus vectors to transduce HIV-1 genes into human dendritic cells". Journal of Medical Virology. 80 (3): 373–382. doi:10.1002/jmv.21052. ISSN 1096-9071. PMID 18205221.
- Nagai, Yoshiyuki (2014-01-31). Sendai virus vector : advantages and applications. Nagai, Yoshiyuki. Tokyo. ISBN 9784431545569. OCLC 870271420.
- Nakanishi, Mahito; Otsu, Makoto (October 2012). "Development of Sendai virus vectors and their potential applications in gene therapy and regenerative medicine". Current Gene Therapy. 12 (5): 410–416. ISSN 1875-5631. PMC 3504922. PMID 22920683.
- Sugiyama, Masahiko; Kakeji, Yoshihiro; Tsujitani, Shunichi; Harada, Yui; Onimaru, Mitsuho; Yoshida, Kumi; Tanaka, Sakura; Emi, Yasunori; Morita, Masaru (March 2011). "Antagonism of VEGF by genetically engineered dendritic cells is essential to induce antitumor immunity against malignant ascites". Molecular Cancer Therapeutics. 10 (3): 540–549. doi:10.1158/1535-7163.MCT-10-0479. ISSN 1538-8514. PMID 21209070.
- Yoshizaki, Mariko; Hironaka, Takashi; Iwasaki, Hitoshi; Ban, Hiroshi; Tokusumi, Yumiko; Iida, Akihiro; Nagai, Yoshiyuki; Hasegawa, Mamoru; Inoue, Makoto (September 2006). "Naked Sendai virus vector lacking all of the envelope-related genes: reduced cytopathogenicity and immunogenicity". The Journal of Gene Medicine. 8 (9): 1151–1159. doi:10.1002/jgm.938. ISSN 1099-498X. PMID 16841365.
- Hasegawa, Mamoru; Iida, Akihiro; Nagai, Yoshiyuki; Hirata, Takahiro; Tokusumi, Tsuyoshi; Shirakura, Masayuki; Kanaya, Takumi; Ban, Hiroshi; Tokusumi, Yumiko (2003-06-01). "A New Sendai Virus Vector Deficient in the Matrix Gene Does Not Form Virus Particles and Shows Extensive Cell-to-Cell Spreading". Journal of Virology. 77 (11): 6419–6429. doi:10.1128/JVI.77.11.6419-6429.2003. ISSN 0022-538X. PMC 155001. PMID 12743299.
- Burke, Crystal W.; Mason, John N.; Surman, Sherri L.; Jones, Bart G.; Dalloneau, Emilie; Hurwitz, Julia L.; Russell, Charles J. (2011-07-07). "Illumination of Parainfluenza Virus Infection and Transmission in Living Animals Reveals a Tissue-Specific Dichotomy". PLOS Pathogens. 7 (7): e1002134. doi:10.1371/journal.ppat.1002134. ISSN 1553-7366. PMC 3131265. PMID 21750677.
- Mostafa, Heba H.; Vogel, Peter; Srinivasan, Ashok; Russell, Charles J. (2016). "Non-invasive Imaging of Sendai Virus Infection in Pharmacologically Immunocompromised Mice: NK and T Cells, but not Neutrophils, Promote Viral Clearance after Therapy with Cyclophosphamide and Dexamethasone". PLOS Pathogens. 12 (9): e1005875. doi:10.1371/journal.ppat.1005875. ISSN 1553-7374. PMC 5010285. PMID 27589232.
- Fusaki, Noemi; Ban, Hiroshi; Nishiyama, Akiyo; Saeki, Koichi; Hasegawa, Mamoru (2009). "Efficient induction of transgene-free human pluripotent stem cells using a vector based on Sendai virus, an RNA virus that does not integrate into the host genome". Proceedings of the Japan Academy. Series B, Physical and Biological Sciences. 85 (8): 348–362. Bibcode:2009PJAB...85..348F. doi:10.2183/pjab.85.348. ISSN 1349-2896. PMC 3621571. PMID 19838014.
- Ban, Hiroshi; Nishishita, Naoki; Fusaki, Noemi; Tabata, Toshiaki; Saeki, Koichi; Shikamura, Masayuki; Takada, Nozomi; Inoue, Makoto; Hasegawa, Mamoru (2011-08-23). "Efficient generation of transgene-free human induced pluripotent stem cells (iPSCs) by temperature-sensitive Sendai virus vectors". Proceedings of the National Academy of Sciences of the United States of America. 108 (34): 14234–14239. Bibcode:2011PNAS..10814234B. doi:10.1073/pnas.1103509108. ISSN 1091-6490. PMC 3161531. PMID 21821793.
- Nishikawa, Shin-Ichi; Kawamata, Shin; Hasegawa, Mamoru; Inoue, Makoto; Takada, Nozomi; Shikamura, Masayuki; Saeki, Koichi; Tabata, Toshiaki; Fusaki, Noemi (2011-08-23). "Efficient generation of transgene-free human induced pluripotent stem cells (iPSCs) by temperature-sensitive Sendai virus vectors". Proceedings of the National Academy of Sciences. 108 (34): 14234–14239. Bibcode:2011PNAS..10814234B. doi:10.1073/pnas.1103509108. ISSN 0027-8424. PMID 21821793.
- Fujie, Yasumitsu; Fusaki, Noemi; Katayama, Tomohiko; Hamasaki, Makoto; Soejima, Yumi; Soga, Minami; Ban, Hiroshi; Hasegawa, Mamoru; Yamashita, Satoshi (2014-12-05). "New Type of Sendai Virus Vector Provides Transgene-Free iPS Cells Derived from Chimpanzee Blood". PLOS ONE. 9 (12): e113052. Bibcode:2014PLoSO...9k3052F. doi:10.1371/journal.pone.0113052. ISSN 1932-6203. PMC 4257541. PMID 25479600.
- "CTS CytoTune-iPS 2.1 Sendai Reprogramming Kit - Thermo Fisher Scientific". www.thermofisher.com. Retrieved 2019-08-13.
- "HN - Hemagglutinin-neuraminidase - Sendai virus (strain Z) (SeV) - HN gene & protein". www.uniprot.org. Retrieved 2019-08-09.
- Scheid A, Choppin PW (February 1974). "Identification of biological activities of paramyxovirus glycoproteins. Activation of cell fusion, hemolysis, and infectivity of proteolytic cleavage of an inactive precursor protein of Sendai virus". Virology. 57 (2): 475–90. doi:10.1016/0042-6822(74)90187-1. PMID 4361457.
- "F - Fusion glycoprotein F0 precursor - Sendai virus (strain Z) (SeV) - F gene & protein". www.uniprot.org. Retrieved 2019-08-09.
- "M - Matrix protein - Sendai virus (strain Ohita) (SeV) - M gene & protein". www.uniprot.org. Retrieved 2019-08-09.
- "N - Nucleoprotein - Sendai virus (strain Z) (SeV) - N gene & protein". www.uniprot.org. Retrieved 2019-08-09.
- "P/V/C - Phosphoprotein - Sendai virus (strain Harris) (SeV) - P/V/C gene & protein". www.uniprot.org. Retrieved 2019-08-09.
- "L - RNA-directed RNA polymerase L - Sendai virus (strain Enders) (SeV) - L gene & protein". www.uniprot.org. Retrieved 2019-08-09.
- Yamada, H.; Hayata, S.; Omata-Yamada, T.; Taira, H.; Mizumoto, K.; Iwasaki, K. (1990). "Association of the Sendai virus C protein with nucleocapsids". Archives of Virology. 113 (3–4): 245–253. doi:10.1007/bf01316677. ISSN 0304-8608. PMID 2171459.
- Faísca, P.; Desmecht, D. (February 2007). "Sendai virus, the mouse parainfluenza type 1: A longstanding pathogen that remains up-to-date". Research in Veterinary Science. 82 (1): 115–125. doi:10.1016/j.rvsc.2006.03.009. ISSN 0034-5288. PMID 16759680.
- Portner, A.; Scroggs, R.A.; Naeve, C.W. (April 1987). "The fusion glycoprotein of Sendai virus: Sequence analysis of an epitope involved in fusion and virus neutralization". Virology. 157 (2): 556–559. doi:10.1016/0042-6822(87)90301-1. ISSN 0042-6822. PMID 2435061.
- Payne, V.; Kam, P. C. A. (July 2004). "Mast cell tryptase: a review of its physiology and clinical significance". Anaesthesia. 59 (7): 695–703. doi:10.1111/j.1365-2044.2004.03757.x. ISSN 0003-2409. PMID 15200544.
- Chen Y, Shiota M, Ohuchi M, Towatari T, Tashiro J, Murakami M, et al. (June 2000). "Mast cell tryptase from pig lungs triggers infection by pneumotropic Sendai and influenza A viruses. Purification and characterization". European Journal of Biochemistry. 267 (11): 3189–97. doi:10.1046/j.1432-1327.2000.01346.x. PMID 10824103.
- Tashiro M, Yokogoshi Y, Tobita K, Seto JT, Rott R, Kido H (December 1992). "Tryptase Clara, an activating protease for Sendai virus in rat lungs, is involved in pneumopathogenicity". Journal of Virology. 66 (12): 7211–6. PMC 240423. PMID 1331518.
- Kido H, Niwa Y, Beppu Y, Towatari T (1996). "Cellular proteases involved in the pathogenicity of enveloped animal viruses, human immunodeficiency virus, influenza virus A and Sendai virus". Advances in Enzyme Regulation. 36: 325–47. doi:10.1016/0065-2571(95)00016-X. PMID 8869754.
- Le TQ, Kawachi M, Yamada H, Shiota M, Okumura Y, Kido H (April 2006). "Identification of trypsin I as a candidate for influenza A virus and Sendai virus envelope glycoprotein processing protease in rat brain". Biological Chemistry. 387 (4): 467–75. doi:10.1515/BC.2006.062. PMID 16606346.
- Murakami M, Towatari T, Ohuchi M, Shiota M, Akao M, Okumura Y, et al. (May 2001). "Mini-plasmin found in the epithelial cells of bronchioles triggers infection by broad-spectrum influenza A viruses and Sendai virus". European Journal of Biochemistry. 268 (10): 2847–55. doi:10.1046/j.1432-1327.2001.02166.x. PMID 11358500.
- Abe M, Tahara M, Sakai K, Yamaguchi H, Kanou K, Shirato K, et al. (November 2013). "TMPRSS2 is an activating protease for respiratory parainfluenza viruses". Journal of Virology. 87 (21): 11930–5. doi:10.1128/JVI.01490-13. PMC 3807344. PMID 23966399.
- Gotoh, B.; Ogasawara, T.; Toyoda, T.; Inocencio, N. M.; Hamaguchi, M.; Nagai, Y. (December 1990). "An endoprotease homologous to the blood clotting factor X as a determinant of viral tropism in chick embryo". The EMBO Journal. 9 (12): 4189–4195. doi:10.1002/j.1460-2075.1990.tb07643.x. ISSN 0261-4189. PMC 552195. PMID 2174359.
- Ogasawara, T; Gotoh, B; Suzuki, H; Asaka, J; Shimokata, K; Rott, R; Nagai, Y (February 1992). "Expression of factor X and its significance for the determination of paramyxovirus tropism in the chick embryo". The EMBO Journal. 11 (2): 467–472. doi:10.1002/j.1460-2075.1992.tb05076.x. ISSN 0261-4189. PMC 556476. PMID 1371460.
- Gotoh, B.; Yamauchi, F.; Ogasawara, T.; Nagai, Y. (1992-01-27). "Isolation of factor Xa from chick embryo as the amniotic endoprotease responsible for paramyxovirus activation". FEBS Letters. 296 (3): 274–278. doi:10.1016/0014-5793(92)80303-x. ISSN 0014-5793. PMID 1537403.
- Sakai Y, Kiyotani K, Fukumura M, Asakawa M, Kato A, Shioda T, et al. (August 1999). "Accommodation of foreign genes into the Sendai virus genome: sizes of inserted genes and viral replication". FEBS Letters. 456 (2): 221–6. doi:10.1016/s0014-5793(99)00960-6. PMID 10456313.
- Curran J, Kolakofsky D (January 1988). "Ribosomal initiation from an ACG codon in the Sendai virus P/C mRNA". The EMBO Journal. 7 (1): 245–51. doi:10.1002/j.1460-2075.1988.tb02806.x. PMC 454264. PMID 2834203.
- Dillon PJ, Gupta KC (February 1989). "Expression of five proteins from the Sendai virus P/C mRNA in infected cells". Journal of Virology. 63 (2): 974–7. PMC 247778. PMID 2536120.
- de Breyne S, Simonet V, Pelet T, Curran J (January 2003). "Identification of a cis-acting element required for shunt-mediated translational initiation of the Sendai virus Y proteins". Nucleic Acids Research. 31 (2): 608–18. doi:10.1093/nar/gkg143. PMC 140508. PMID 12527769.
- Kolakofsky, Daniel; Itoh, Masae; Curran, Joseph; Garcin, Dominique (2001-08-01). "Longer and Shorter Forms of Sendai Virus C Proteins Play Different Roles in Modulating the Cellular Antiviral Response". Journal of Virology. 75 (15): 6800–6807. doi:10.1128/JVI.75.15.6800-6807.2001. ISSN 0022-538X. PMC 114406. PMID 11435558.
- Curran, J.; Kolakofsky, D. (September 1988). "Scanning independent ribosomal initiation of the Sendai virus X protein". The EMBO Journal. 7 (9): 2869–2874. doi:10.1002/j.1460-2075.1988.tb03143.x. ISSN 0261-4189. PMC 457080. PMID 2846286.
- Irie, Takashi; Nagata, Natsuko; Yoshida, Tetsuya; Sakaguchi, Takemasa (2008-02-05). "Recruitment of Alix/AIP1 to the plasma membrane by Sendai virus C protein facilitates budding of virus-like particles". Virology. 371 (1): 108–120. doi:10.1016/j.virol.2007.09.020. ISSN 0042-6822. PMID 18028977.
- Clark, Georgina; Zola, Heddy; Zelm, Menno C. van; Stockinger, Hannes; Schwartz-Albiez, Reinhard; Mortari, Frank; Malavasi, Fabio; Jin, Bo-Quan; Horejsi, Vaclav (2015-11-15). "CD Nomenclature 2015: Human Leukocyte Differentiation Antigen Workshops as a Driving Force in Immunology". The Journal of Immunology. 195 (10): 4555–4563. doi:10.4049/jimmunol.1502033. ISSN 0022-1767. PMID 26546687.
- Bitzer, M; Lauer, U; Baumann, C; Spiegel, M; Gregor, M; Neubert, W J (July 1997). "Sendai virus efficiently infects cells via the asialoglycoprotein receptor and requires the presence of cleaved F0 precursor proteins for this alternative route of cell entry". Journal of Virology. 71 (7): 5481–5486. ISSN 0022-538X. PMC 191789. PMID 9188621.
- Suzuki Y, Suzuki T, Matsumoto M (June 1983). "Isolation and characterization of receptor sialoglycoprotein for hemagglutinating virus of Japan (Sendai virus) from bovine erythrocyte membrane". Journal of Biochemistry. 93 (6): 1621–33. doi:10.1093/oxfordjournals.jbchem.a134301. PMID 6309760.
- Oku N, Nojima S, Inoue K (August 1981). "Studies on the interaction of Sendai virus with liposomal membranes. Sendai virus-induced agglutination of liposomes containing glycophorin". Biochimica et Biophysica Acta. 646 (1): 36–42. doi:10.1016/0005-2736(81)90269-8. PMID 6168285.
- Müthing J (September 1996). "Influenza A and Sendai viruses preferentially bind to fucosylated gangliosides with linear poly-N-acetyllactosaminyl chains from human granulocytes". Carbohydrate Research. 290 (2): 217–24. doi:10.1016/0008-6215(96)00149-8. PMID 8823909.
- "KEGG GLYCAN: G00197". www.genome.jp. Retrieved 2019-08-13.
- Villar E, Barroso IM (February 2006). "Role of sialic acid-containing molecules in paramyxovirus entry into the host cell: a minireview". Glycoconjugate Journal. 23 (1–2): 5–17. doi:10.1007/s10719-006-5433-0. PMID 16575518.
- Holmgren J, Svennerholm L, Elwing H, Fredman P, Strannegård O (April 1980). "Sendai virus receptor: proposed recognition structure based on binding to plastic-adsorbed gangliosides". Proceedings of the National Academy of Sciences of the United States of America. 77 (4): 1947–50. Bibcode:1980PNAS...77.1947H. doi:10.1073/pnas.77.4.1947. PMC 348626. PMID 6246515.
- "Correction: Specific Gangliosides Function as Host Cell Receptors for Sendai Virus". Proceedings of the National Academy of Sciences. 79 (3): 951. 1982-02-01. Bibcode:1982PNAS...79Q.951.. doi:10.1073/pnas.79.3.951.
- Suzuki Y, Suzuki T, Matsunaga M, Matsumoto M (April 1985). "Gangliosides as paramyxovirus receptor. Structural requirement of sialo-oligosaccharides in receptors for hemagglutinating virus of Japan (Sendai virus) and Newcastle disease virus". Journal of Biochemistry. 97 (4): 1189–99. doi:10.1093/oxfordjournals.jbchem.a135164. PMID 2993261.
- Suzuki, Takashi; Portner, Allen; Scroggs, Ruth Ann; Uchikawa, Makoto; Koyama, Noriko; Matsuo, Kazuko; Suzuki, Yasuo; Takimoto, Toru (May 2001). "Receptor Specificities of Human Respiroviruses". Journal of Virology. 75 (10): 4604–4613. doi:10.1128/JVI.75.10.4604-4613.2001. ISSN 0022-538X. PMC 114213. PMID 11312330.
- "KEGG GLYCAN: G00116". www.genome.jp. Retrieved 2019-08-13.
- "KEGG GLYCAN: G00112". www.genome.jp. Retrieved 2019-08-13.
- "KEGG GLYCAN: G00122". www.genome.jp. Retrieved 2019-08-13.
- "KEGG GLYCAN: G00117". www.genome.jp. Retrieved 2019-08-13.
- Umeda M, Nojima S, Inoue K (February 1984). "Activity of human erythrocyte gangliosides as a receptor to HVJ". Virology. 133 (1): 172–82. doi:10.1016/0042-6822(84)90436-7. PMID 6322427.
- Suzuki T, Portner A, Scroggs RA, Uchikawa M, Koyama N, Matsuo K, et al. (May 2001). "Receptor specificities of human respiroviruses". Journal of Virology. 75 (10): 4604–13. doi:10.1128/jvi.75.10.4604-4613.2001. PMC 114213. PMID 11312330.
- Mariethoz, Julien; Khatib, Khaled; Campbell, Matthew P.; Packer, Nicolle H.; Mullen, Elaine; Lisacek, Frederique (2014-10-20), "SugarBindDB SugarBindDB : Resource of Pathogen Pathogen Lectin-Glycan Interactions Lectin-glycan interactions", Glycoscience: Biology and Medicine, Springer Japan, pp. 275–282, doi:10.1007/978-4-431-54841-6_28, ISBN 9784431548409
- "DBGET search - GLYCAN". www.genome.jp. Retrieved 2019-08-15.
- "About PubChem". pubchemdocs.ncbi.nlm.nih.gov. Retrieved 2019-08-15.
- "TOXNET". toxnet.nlm.nih.gov. Retrieved 2019-08-15.
- Sterner, Eric; Flanagan, Natalie; Gildersleeve, Jeffrey C. (2016-05-25). "Perspectives on Anti-Glycan Antibodies Gleaned from Development of a Community Resource Database". ACS Chemical Biology. 11 (7): 1773–1783. doi:10.1021/acschembio.6b00244. ISSN 1554-8929. PMC 4949583. PMID 27220698.
- Britten, Christopher J; Bird, Michael I (February 1997). "Chemical modification of an α3-fucosyltransferase; definition of amino acid residues essential for enzyme activity". Biochimica et Biophysica Acta (BBA) - General Subjects. 1334 (1): 57–64. doi:10.1016/s0304-4165(96)00076-1. ISSN 0304-4165. PMID 9042366.
- de Vries, T.; Knegtel, R. M.A.; Holmes, E. H.; Macher, B. A. (2001-10-01). "Fucosyltransferases: structure/function studies". Glycobiology. 11 (10): 119R–128R. doi:10.1093/glycob/11.10.119r. ISSN 0959-6658. PMID 11588153.
- Shetterly, Susan; Jost, Franziska; Watson, Susan R.; Knegtel, Ronald; Macher, Bruce A.; Holmes, Eric H. (2007-06-29). "Site-specific Fucosylation of Sialylated Polylactosamines by α1,3/4-Fucosyltransferases-V and -VI Is Defined by Amino Acids Near the N Terminus of the Catalytic Domain". Journal of Biological Chemistry. 282 (34): 24882–24892. doi:10.1074/jbc.m702395200. ISSN 0021-9258. PMID 17604274.
- Trinchera, Marco; Aronica, Adele; Dall’Olio, Fabio (2017-02-23). "Selectin Ligands Sialyl-Lewis a and Sialyl-Lewis x in Gastrointestinal Cancers". Biology. 6 (4): 16. doi:10.3390/biology6010016. ISSN 2079-7737. PMC 5372009. PMID 28241499.
- Ngamukote, Sathaporn; Yanagisawa, Makoto; Ariga, Toshio; Ando, Susumu; Yu, Robert K. (December 2007). "Developmental changes of glycosphingolipids and expression of glycogenes in mouse brains". Journal of Neurochemistry. 103 (6): 2327–2341. doi:10.1111/j.1471-4159.2007.04910.x. ISSN 0022-3042. PMID 17883393.
- Xu, Weiming; Kozak, Christine A.; Desnick, Robert J. (April 1995). "Uroporphyrinogen-III synthase: molecular cloning, nucleotide sequence, expression of a mouse full-length cDNA, and its localization on mouse chromosome 7". Genomics. 26 (3): 556–562. doi:10.1016/0888-7543(95)80175-l. ISSN 0888-7543. PMID 7607680.
- Vandermeersch, Sandy; Vanbeselaere, Jorick; Delannoy, Clément; Drolez, Aurore; Mysiorek, Caroline; Guérardel, Yann; Delannoy, Philippe; Julien, Sylvain (2015-04-16). "Accumulation of GD1α Ganglioside in MDA-MB-231 Breast Cancer Cells Expressing ST6GalNAc V". Molecules. 20 (4): 6913–6924. doi:10.3390/molecules20046913. ISSN 1420-3049. PMC 6272744. PMID 25913930.
- Chandrasekaran, E. V.; Xue, Jun; Xia, Jie; Locke, Robert D.; Patil, Shilpa A.; Neelamegham, Sriram; Matta, Khushi L. (2011-11-08). "Mammalian Sialyltransferase ST3Gal-II: Its Exchange Sialylation Catalytic Properties Allow Labeling of Sialyl Residues in Mucin-Type Sialylated Glycoproteins and Specific Gangliosides". Biochemistry. 50 (44): 9475–9487. doi:10.1021/bi200301w. ISSN 0006-2960. PMC 3206213. PMID 21913655.
- Rawling J, Cano O, Garcin D, Kolakofsky D, Melero JA (March 2011). "Recombinant Sendai viruses expressing fusion proteins with two furin cleavage sites mimic the syncytial and receptor-independent infection properties of respiratory syncytial virus". Journal of Virology. 85 (6): 2771–80. doi:10.1128/JVI.02065-10. PMC 3067931. PMID 21228237.
- Hoekstra D, Klappe K, Hoff H, Nir S (April 1989). "Mechanism of fusion of Sendai virus: role of hydrophobic interactions and mobility constraints of viral membrane proteins. Effects of polyethylene glycol". The Journal of Biological Chemistry. 264 (12): 6786–92. PMID 2540161.
- Takimoto T, Taylor GL, Connaris HC, Crennell SJ, Portner A (December 2002). "Role of the hemagglutinin-neuraminidase protein in the mechanism of paramyxovirus-cell membrane fusion". Journal of Virology. 76 (24): 13028–33. doi:10.1128/JVI.76.24.13028-13033.2002. PMC 136693. PMID 12438628.
- Novick SL, Hoekstra D (October 1988). "Membrane penetration of Sendai virus glycoproteins during the early stages of fusion with liposomes as determined by hydrophobic photoaffinity labeling". Proceedings of the National Academy of Sciences of the United States of America. 85 (20): 7433–7. Bibcode:1988PNAS...85.7433N. doi:10.1073/pnas.85.20.7433. PMC 282205. PMID 2845406.
- Keskinen, P.; Nyqvist, M.; Sareneva, T.; Pirhonen, J.; Melén, K.; Julkunen, I. (1999-10-25). "Impaired antiviral response in human hepatoma cells". Virology. 263 (2): 364–375. doi:10.1006/viro.1999.9983. ISSN 0042-6822. PMID 10544109.
- Shah NR, Sunderland A, Grdzelishvili VZ (June 2010). "Cell type mediated resistance of vesicular stomatitis virus and Sendai virus to ribavirin". PLOS ONE. 5 (6): e11265. Bibcode:2010PLoSO...511265S. doi:10.1371/journal.pone.0011265. PMC 2889835. PMID 20582319.
- Sumpter R, Loo YM, Foy E, Li K, Yoneyama M, Fujita T, et al. (March 2005). "Regulating intracellular antiviral defense and permissiveness to hepatitis C virus RNA replication through a cellular RNA helicase, RIG-I". Journal of Virology. 79 (5): 2689–99. doi:10.1128/JVI.79.5.2689-2699.2005. PMC 548482. PMID 15708988.
- Lallemand C, Blanchard B, Palmieri M, Lebon P, May E, Tovey MG (January 2007). "Single-stranded RNA viruses inactivate the transcriptional activity of p53 but induce NOXA-dependent apoptosis via post-translational modifications of IRF-1, IRF-3 and CREB". Oncogene. 26 (3): 328–38. doi:10.1038/sj.onc.1209795. PMID 16832344.
- Buggele WA, Horvath CM (August 2013). "MicroRNA profiling of Sendai virus-infected A549 cells identifies miR-203 as an interferon-inducible regulator of IFIT1/ISG56". Journal of Virology. 87 (16): 9260–70. doi:10.1128/JVI.01064-13. PMC 3754065. PMID 23785202.
- Abe, Masako; Tahara, Maino; Sakai, Kouji; Yamaguchi, Hiromi; Kanou, Kazuhiko; Shirato, Kazuya; Kawase, Miyuki; Noda, Masahiro; Kimura, Hirokazu (November 2013). "TMPRSS2 Is an Activating Protease for Respiratory Parainfluenza Viruses". Journal of Virology. 87 (21): 11930–11935. doi:10.1128/JVI.01490-13. ISSN 0022-538X. PMC 3807344. PMID 23966399.
- Zainutdinov SS, Grazhdantseva AA, Kochetkov DV, Chumakov PM, Netesov SV, Matveeva OV, Kochneva GV (2017-10-01). "Change in Oncolytic Activity of Sendai Virus during Adaptation to Cell Cultures". Molecular Genetics, Microbiology and Virology. 32 (4): 212–217. doi:10.3103/S0891416817040115.
- Mandhana R, Horvath CM (November 2018). "Sendai Virus Infection Induces Expression of Novel RNAs in Human Cells". Scientific Reports. 8 (1): 16815. Bibcode:2018NatSR...816815M. doi:10.1038/s41598-018-35231-8. PMC 6235974. PMID 30429577.
- Belova, A. A.; Sosnovtseva, A. O.; Lipatova, A. V.; Njushko, K. M.; Volchenko, N. N.; Belyakov, M. M.; Sudalenko, O. V.; Krasheninnikov, A. A.; Shegai, P. V. (2017-01-01). "Biomarkers of prostate cancer sensitivity to the Sendai virus". Molecular Biology. 51 (1): 80–88. doi:10.1134/S0026893317010046. ISSN 1608-3245.
- Power, Ultan F.; Shields, Michael D.; Coyle, Peter V.; McKaigue, James P.; Heaney, Liam G.; Skibinski, Grzegorz; Parker, Jeremy; Sarlang, Severine; Thavagnanam, Surendran (2010-11-15). "Cytopathogenesis of Sendai Virus in Well-Differentiated Primary Pediatric Bronchial Epithelial Cells". Journal of Virology. 84 (22): 11718–11728. doi:10.1128/JVI.00798-10. ISSN 0022-538X. PMC 2977906. PMID 20810726.
- Burke CW, Mason JN, Surman SL, Jones BG, Dalloneau E, Hurwitz JL, Russell CJ (July 2011). "Illumination of parainfluenza virus infection and transmission in living animals reveals a tissue-specific dichotomy". PLOS Pathogens. 7 (7): e1002134. doi:10.1371/journal.ppat.1002134. PMC 3131265. PMID 21750677.
- Itoh, M.; Wang, X. L.; Suzuki, Y.; Homma, M. (September 1992). "Mutation of the HANA protein of Sendai virus by passage in eggs". Virology. 190 (1): 356–364. doi:10.1016/0042-6822(92)91222-g. ISSN 0042-6822. PMID 1326808.
- Zainutdinov SS, Kochneva GV, Netesov SV, Chumakov PM, Matveeva OV (2019). "Directed evolution as a tool for the selection of oncolytic RNA viruses with desired phenotypes". Oncolytic Virotherapy. 8: 9–26. doi:10.2147/OV.S176523. PMC 6636189. PMID 31372363.
- Itoh M, Wang XL, Suzuki Y, Homma M (September 1992). "Mutation of the HANA protein of Sendai virus by passage in eggs". Virology. 190 (1): 356–64. doi:10.1016/0042-6822(92)91222-g. PMID 1326808.
- Sakaguchi T, Kiyotani K, Sakaki M, Fujii Y, Yoshida T (March 1994). "A field isolate of Sendai virus: its high virulence to mice and genetic divergence form prototype strains". Archives of Virology. 135 (1–2): 159–64. doi:10.1007/bf01309773. PMID 8198441.
- Itoh, M; Homma, M; Isegawa, Y; Hotta, H (1997-12-01). "Isolation of an avirulent mutant of Sendai virus with two amino acid mutations from a highly virulent field strain through adaptation to LLC-MK2 cells". Journal of General Virology. 78 (12): 3207–3215. doi:10.1099/0022-1317-78-12-3207. ISSN 0022-1317. PMID 9400971.
- Kiyotani, Katsuhiro; Takao, Shinichi; Sakaguchi, Takemasa; Yoshida, Tetsuya (July 1990). "Immediate protection of mice from lethal wild-type Sendai virus (HVJ) infections by a temperature-sensitive mutant, HVJpi, possessing homologous interfering capacity". Virology. 177 (1): 65–74. doi:10.1016/0042-6822(90)90460-9. ISSN 0042-6822. PMID 2162116.
- Zhdanov, V. M.; Bukrinskaya, A. G. (1961). "Autoradiographic study of the penetration of Sendai virus into tissue culture cells. I. Preparation of Sendai virus labelled with radioactive isotopes". Problems of Virology. 6: 588–593. ISSN 0555-2621. PMID 14040447.
- Shi LY, Li M, Yuan LJ, Wang Q, Li XM (2008). "A new paramyxovirus, Tianjin strain, isolated from common cotton-eared marmoset: genome characterization and structural protein sequence analysis". Archives of Virology. 153 (9): 1715–23. doi:10.1007/s00705-008-0184-9. PMID 18696006.
- Yang Y, Ren L, Dong X, Wu X (2005-05-01). "108. The Complete Nucleotide Sequence of Sendai Virus Isolate BB1 and Comparison with Other Isolates". Molecular Therapy. 11: S44. doi:10.1016/j.ymthe.2005.06.469.
- Kiyotani, K.; Sakaguchi, T.; Fujii, Y.; Yoshida, T. (2001). "Attenuation of a field Sendai virus isolate through egg-passages is associated with an impediment of viral genome replication in mouse respiratory cells". Archives of Virology. 146 (5): 893–908. doi:10.1007/s007050170123. ISSN 0304-8608. PMID 11448028.
- Kolakofsky, D. (August 1976). "Isolation and characterization of Sendai virus DI-RNAs". Cell. 8 (4): 547–555. doi:10.1016/0092-8674(76)90223-3. ISSN 0092-8674. PMID 182384.
- Mercado-López, Xiomara; Cotter, Christopher R.; Kim, Won-keun; Sun, Yan; Muñoz, Luis; Tapia, Karla; López, Carolina B. (2013-11-19). "Highly Immunostimulatory RNA Derived from a Sendai Virus Defective Viral Genome". Vaccine. 31 (48): 5713–5721. doi:10.1016/j.vaccine.2013.09.040. ISSN 0264-410X. PMC 4406099. PMID 24099876.
- Genoyer, Emmanuelle; López, Carolina B. (2019-02-05). "Defective Viral Genomes Alter How Sendai Virus Interacts with Cellular Trafficking Machinery, Leading to Heterogeneity in the Production of Viral Particles among Infected Cells". Journal of Virology. 93 (4). doi:10.1128/JVI.01579-18. ISSN 0022-538X. PMC 6364009. PMID 30463965.
- Yoshida, Asuka; Kawabata, Ryoko; Honda, Tomoyuki; Sakai, Kouji; Ami, Yasushi; Sakaguchi, Takemasa; Irie, Takashi (March 2018). "A Single Amino Acid Substitution within the Paramyxovirus Sendai Virus Nucleoprotein Is a Critical Determinant for Production of Interferon-Beta-Inducing Copyback-Type Defective Interfering Genomes". Journal of Virology. 92 (5). doi:10.1128/JVI.02094-17. ISSN 1098-5514. PMC 5809723. PMID 29237838.
- Strahle, Laura; Garcin, Dominique; Kolakofsky, Daniel (2006-07-20). "Sendai virus defective-interfering genomes and the activation of interferon-beta". Virology. 351 (1): 101–111. doi:10.1016/j.virol.2006.03.022. ISSN 0042-6822. PMID 16631220.
- Tatsumoto N, Arditi M, Yamashita M (September 2018). "Sendai Virus Propagation Using Chicken Eggs". Bio-Protocol. 8 (18). doi:10.21769/BioProtoc.3009. PMC 6200407. PMID 30370318.
- Donis, Ruben O.; Swayne, David E.; Matsuoka, Yumiko; Pappas, Claudia (2007-11-01). "Development and Evaluation of an Influenza Virus Subtype H7N2 Vaccine Candidate for Pandemic Preparedness". Clinical and Vaccine Immunology. 14 (11): 1425–1432. doi:10.1128/CVI.00174-07. ISSN 1556-6811. PMC 2168170. PMID 17913860.
- Tatsumoto, Narihito; Miyauchi, Takamasa; Arditi, Moshe; Yamashita, Michifumi (2018-11-05). "Quantification of Infectious Sendai Virus Using Plaque Assay". Bio-Protocol. 8 (21). doi:10.21769/BioProtoc.3068. ISSN 2331-8325. PMC 6289198. PMID 30547053.
- Killian, Mary Lea (2008). "Hemagglutination assay for the avian influenza virus". Methods in Molecular Biology. 436: 47–52. doi:10.1007/978-1-59745-279-3_7. ISBN 978-1-58829-939-0. ISSN 1064-3745. PMID 18370040.
- "Sendai virus ATCC ® VR-105™". www.atcc.org. Retrieved 2019-08-14.
- "Sendai virus ATCC ® VR-907™". www.atcc.org. Retrieved 2019-08-14.
- "PTA-121432". www.atcc.org. Retrieved 2019-08-14.
Further reading
- Simon AY, Moritoh K, Torigoe D, Asano A, Sasaki N, Agui T (December 2009). "Multigenic control of resistance to Sendai virus infection in mice". Infection, Genetics and Evolution. 9 (6): 1253–9. doi:10.1016/j.meegid.2009.08.011. hdl:2115/42554. PMID 19733691.
- Faísca, P.; Desmecht, D. (February 2007). "Sendai virus, the mouse parainfluenza type 1: A longstanding pathogen that remains up-to-date". Research in Veterinary Science. 82 (1): 115–125. doi:10.1016/j.rvsc.2006.03.009. ISSN 0034-5288. PMID 16759680.