Cable bacteria
Cable bacteria are filamentous bacteria that conduct electricity across distances over 1 cm in sediment and groundwater aquifers.[1][2] Cable bacteria couple the reduction of oxygen[2] or nitrate[3] at the sediment's surface to the oxidation of sulfide[2] in the deeper, anoxic, sediment layers.
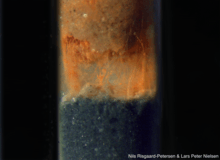
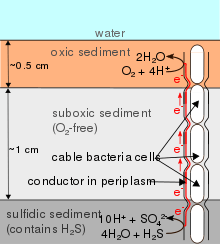
Discovery
Long-distance electrical conductance in sediment was first observed in 2010 as a spatial separation of sulfide oxidation and oxygen reduction in marine sediment that was interrupted and re-established at a rate faster than could be explained by chemical diffusion.[1] It was later found that this electrical conductance could be observed across a non-conductive layer of glass microspheres, where the only possible conductive structures were filamentous bacteria belonging to the family Desulfobulbaceae.[2] The conductivity of single, live filaments was later demonstrated by observing the oxidation state of cytochromes using Raman microscopy.[4] The same phenomenon was later observed in freshwater sediments[5] and groundwater aquifers.[6] Cable bacteria densities of up to 2 km per square centimeter of sediment surface have been observed.[7]
Morphology
Cable bacteria filaments are 0.4—1.7 µm in diameter and up to 15 mm long.[7] Filaments consist of rod-shaped cells with an average length of 3 µm and 15-58 ridges around encircling the cells diameter. These ridges are hypothesized to contain the cells' conductive structures.[2][8] Each ridge contains two parallel "strings" - continuous structures that connect cells in the filament to one another. [9] The diameter of junctions between cells in the filament varies from being smaller than the cell diameter, the same diameter as the cells on either side of the junction, or bulging out to become wider than the cell diameter. [9]
Taxonomy
Two candidate genera of cable bacteria have been described: Electrothrix containing four candidate species, found in marine or brackish sediments, and Electronema containing two candidate species, found in freshwater sediments.[10] These genera are classified within the family Desulfobulbaceae. Cable bacteria are defined by their function rather than their phylogeny, and it is possible that further cable bacteria taxa will be discovered.
Ecological significance
Cable bacteria strongly influence the geochemical properties of the surrounding environment. Their activity promotes the oxidation of iron at the surface of the sediment, and the resulting iron oxides bind phosphorus-containing compounds[11] and hydrogen sulfide,[12] limiting the amount of phosphorus and hydrogen sulfide in the water. Phosphorus can cause eutrophication, and hydrogen sulfide can be toxic to marine life, meaning that cable bacteria play an important role in maintaining marine ecosystems in coastal areas.
Practical applications
Cable bacteria have been found associated with benthic microbial fuel cells, devices that convert chemical energy on the ocean floor to electrical energy.[13] In the future, cable bacteria may play a role in increasing the efficiency of microbial fuel cells. Cable bacteria have also been found associated with a bioelectrochemical system for degrading contaminating hydrocarbons in marine sediment [14] and thus may play a role in future oil spill cleanup technologies.
Extent
Cable bacteria have been identified in a diverse range of climatic conditions worldwide,[15] including Denmark,[2][5] the Netherlands,[8] Japan,[10] Australia,[16] and the United States.[17]
See also
- Biodegradable electronics
References
- Nielsen LP, Risgaard-Petersen N, Fossing H, Christensen PB, Sayama M. (2010). Electric currents couple spatially separated biogeochemical processes in marine sediment. Nature 463: 1071–1074. doi:10.1038/nature08790
- Pfeffer C, Larsen S, Song J, Dong M, Besenbacher F, Meyer RL, et al. (2012). Filamentous bacteria transport electrons over centimetre distances. Nature 491: 218–221. doi:10.1038/nature11586
- Marzocchi U, Trojan D, Larsen S, Meyer RL, Revsbech NP, Schramm A, et al. (2014). Electric coupling between distant nitrate reduction and sulfide oxidation in marine sediment. The ISME Journal 8: 1682–1690. doi:10.1038/ismej.2014.19
- Bjerg JT, Boschker HTS, Larsen S, Berry D, Schmid M, Millo D, et al. (2018). Long-distance electron transport in individual, living cable bacteria. Proc Natl Acad Sci USA 115: 5786–5791. doi:10.1073/pnas.1800367115
- Risgaard-Petersen N, Kristiansen M, Frederiksen RB, Dittmer AL, Bjerg JT, Trojan D, et al. (2015). Cable Bacteria in Freshwater Sediments. Appl Environ Microbiol 81: 6003–6011. doi:10.1128/AEM.01064-15
- Müller H, Bosch J, Griebler C, Damgaard LR, Nielsen LP, Lueders T, et al. (2016). Long-distance electron transfer by cable bacteria in aquifer sediments. The ISME Journal 10: 2010–2019. doi:10.1038/ismej.2015.250
- Schauer R, Risgaard-Petersen N, Kjeldsen KU, Tataru Bjerg JJ, B Jørgensen B, Schramm A, et al. (2014). Succession of cable bacteria and electric currents in marine sediment. The ISME Journal 8: 1314–1322.
- Malkin SY, Rao AMF, Seitaj D, Vasquez-Cardenas D, Zetsche E-M, Hidalgo-Martinez S, et al. (2014). Natural occurrence of microbial sulphur oxidation by long-range electron transport in the seafloor. The ISME Journal 8: 1843–1854. doi:10.1038/ismej.2014.41
- Jiang Z, Zhang S, Klausen LH, Song J, Li Q, Wang Z, et al. (2018). In vitro single-cell dissection revealing the interior structure of cable bacteria. Proc Natl Acad Sci USA 115: 8517–8522.doi:10.1073/pnas.1807562115
- Trojan D, Schreiber L, Bjerg JT, Bøggild A, Yang T, Kjeldsen KU, et al. (2016). A taxonomic framework for cable bacteria and proposal of the candidate genera Electrothrix and Electronema. Syst Appl Microbiol 39: 297–306. doi:10.1016/j.syapm.2016.05.006
- Sulu-Gambari F, Seitaj D, Meysman FJR, Schauer R, Polerecky L, Slomp CP. (2016). Cable Bacteria Control Iron-Phosphorus Dynamics in Sediments of a Coastal Hypoxic Basin. Environ Sci Technol 50: 1227–1233. doi:10.1021/acs.est.5b04369
- Seitaj D, Schauer R, Sulu-Gambari F, Hidalgo-Martinez S, Malkin SY, Burdorf LDW, et al. (2015). Cable bacteria generate a firewall against euxinia in seasonally hypoxic basins. Proc Natl Acad Sci USA 112: 13278–13283. doi:10.1073/pnas.1510152112
- Reimers CE, Li C, Graw MF, Schrader PS, Wolf M. (2017). The Identification of Cable Bacteria Attached to the Anode of a Benthic Microbial Fuel Cell: Evidence of Long Distance Extracellular Electron Transport to Electrodes. Front Microbio 8: 563. doi:10.1101/pdb.prot5366
- Matturro B, Cruz Viggi C, Aulenta F, Rossetti S. (2017). Cable Bacteria and the Bioelectrochemical Snorkel: The Natural and Engineered Facets Playing a Role in Hydrocarbons Degradation in Marine Sediments. Front Microbio 8: 952. doi:10.3389/fmicb.2017.00952
- Burdorf LDW, Tramper A, Seitaj D, Meire L, Hidalgo-Martinez S, Zetsche E-M, et al. (2017). Long-distance electron transport occurs globally in marine sediments. Biogeosciences 14: 683–701. doi:10.5194/bg-14-683-2017
- "Shock as scientists find 'electric' bacteria in the Yarra". 2014-12-05.
- Larsen S, Nielsen LP, Schramm A. (2014). Cable bacteria associated with long-distance electron transport in New England salt marsh sediment. Environmental Microbiology Reports 7: 175–179. doi:10.1111/1758-2229.12216
External links
Media related to Cable bacteria at Wikimedia Commons